Table of Contents
Introduction
Hydrocarbon refining is a process designed to transform crude oil into products of high commercial value, such as fuels, lubricants and petrochemicals. At the core of these industrial processes are reactors, devices that facilitate the chemical transformations necessary to obtain specific products. This article will explore the types most commonly used in the hydrocarbon refining industry, their essential role and the particularities that make them effective in specific applications.
What is a reactor?
In hydrocarbon refining processes, a reactor is a pressurized vessel designed for controlled chemical reactions to take place inside it. These reactions involve chemical transformations in which different substances, known as reactants, interact under specific conditions of temperature, pressure and, in many cases, with the help of catalysts to produce high value-added products. Reactors are fundamental in the refining of hydrocarbons, as they facilitate the manufacture of a wide range of products, such as fuels (gasoline, diesel, kerosene), lubricants and basic chemicals used in various industrial applications.
Types of reactors and their role in hydrocarbon refining processes
There are several types chemical reactors used in hydrocarbon refining, each designed to meet specific needs depending on the type of reaction and the characteristics of the process. The most prominent include:
Fixed bed reactors: They are commonly used in hydrotreating and catalytic reforming processes. In this type of reactor, the catalyst remains stationary while the hydrocarbon flow passes through the bed.
- Advantages: They provide uniform contact between the reagents and the catalyst, which improves conversion and selectivity.
- Applications: Hydrotreating, desulfurization and catalytic reforming.
- Limitations: They can present coke accumulation problems, which affects catalyst activity.
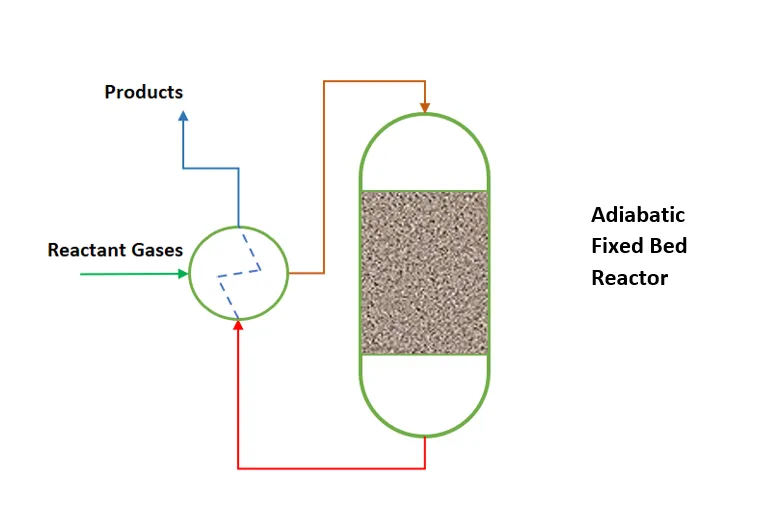
Fluidized bed reactors: In this design, the catalyst is in the form of fine particles that are suspended by an upward flow of gas or liquid. This type of reactor is widely used in catalytic cracking.
- Advantages: Excellent heat and mass transfer, high processability and possibility of continuous catalyst regeneration.
- Applications: Catalytic cracking, alkylation processes.
- Limitations: Requires complex systems for separation of catalyst from product.
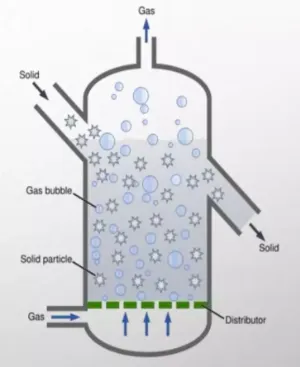
Tubular reactors: They are elongated and of small cross section where the reactants flow through a tube that may be coated with catalyst. They are ideal for endothermic reactions due to their ability to dissipate heat.
- Advantages: Precise temperature control and the ability to handle high pressures.
- Applications: Catalytic reforming and oxidation processes.
- Limitations: High complexity in design and maintenance.
Stirred tank reactors: This type of reactor uses a stirrer to mix the reactants and catalyst, ensuring uniform contact. They are used in processes where homogeneity is crucial.
- Advantages: Flexibility in operation and design, and good heat transfer.
- Applications: Hydrogenation, chemical synthesis.
- Limitations: Limited to small and medium scales.
Stirred tank reactors design scheme4 .
Processes in refining and associated reactors
Catalytic cracking: An endothermic process that breaks large hydrocarbon molecules into smaller fractions, such as gasolines and olefins. For this process, fluidized bed reactors of catalytic particles in continuous motion are used, as they offer high conversion efficiency and allow continuous regeneration of the catalyst.
Hydrotreating: This catalytic process removes impurities such as sulfur, nitrogen and heavy metals from hydrocarbons. Fixed-bed reactors are used because of their ability to handle constant flows of reagents and their ease of operation at high pressures and temperatures.
Hydrocracking: A catalytic process that combines hydrogenation and cracking to convert heavy hydrocarbon fractions into higher value products such as gasoline, diesel and kerosne. These are, process is carried out in fixed-bed reactors operating at high pressures and temperatures. This is reactorare designed to handle constant flows of hydrogen and allow an even distribution of reactants over the catalyst, which ensures high conversion and efficient control of side reactions.
Catalytic Reforming: Catalytic reforming is an endothermic process used to improve the quality of naphtha by increasing the octane number. It is carried out in fixed or continuous bed reactors, where platinum and rhenium-based catalysts are used to promote reactions such as isomerization, dehydrogenation and cyclization of hydrocarbons. These are designed to maintain a uniform temperature and control pressure conditions, ensuring catalyst stability and optimizing the production of high-octane gasoline and aromatic compounds.
Alkylation: Alkylation is an exothermic process that combines light olefins, such as propylene and butylene, with isobutane in the presence of an acid catalyst, such as sulfuric or hydrofluoric acid. The reactors used in this process are of the continuous type, with designs that ensure efficient mixing of the reactants and precise temperature control to avoid unwanted side reactions. This process produces high octane alkylates with low pollutant emissions.
Thermal Coking: Thermal cracking, or coking, is an endothermic process in which heavy hydrocarbons are decomposed at high temperatures without the use of catalysts to produce lighter products, such as naphthas and gas oil. A fluidized bed or tube furnace-type reactor designed to withstand extreme temperatures and minimize coke formation on the walls is used. This process generates coke as a solid by-product, which is separated from the main stream and can be used as an energy source or precursor material for other industries.
Isomerization: Isomerization is a process used to convert linear hydrocarbons into their branched isomers, improving the octane number of gasolines. The reactors used in this process are fixed-bed working under moderate pressure and temperature conditions, with platinum or zeolite-based catalysts. These are ensure a uniform flow of reagents, which optimizes conversion and minimizes the formation of undesirable by-products.
Claus Reactor: The Claus process is used for the recovery of sulfur from hydrogen sulfide (H₂S) rich gases. Claus reactors consist of reaction chambers designed to handle exothermic partial combustion and catalytic conversion reactions. Specific catalysts are employed that maximize elemental sulfur production while ensuring high efficiency in the removal of gaseous contaminants. The reactor design includes cooling and condensation stages to facilitate the separation of the produced sulfur.
Hydrothermal Catalytic Disintegration (H-Oil): Combines hydrotreating and catalytic cracking in a suspended bed reactor. This reactor is designed to handle heavy fractions of crude oil and convert them into lighter products, such as gas oils and distillate fuels. The suspended bed configuration ensures efficient contact between the reactants and the catalyst in the presence of hydrogen, which improves conversion and minimizes coke formation. This design is ideal for handling feeds with high levels of impurities and residues.
Factors influencing reactors selection
The selection of reactors for a specific process depends on several factors, such as:
Nature of the chemical reaction: Catalytic or non-catalytic reaction: If the reaction requires a catalyst, such as fixed bed or fluidized bed reactors are chosen.
Endothermic or exothermic reaction: Processes involving heat transfer, such as catalytic reforming (endothermic) or hydrotreating (exothermic), require reactors with efficient thermal control, such as tube bundle or multi-tube reactors.
Characteristics of reactants and products: Whether the reactants are in liquid, gas or mixed phase influences the reactor design. For example, for gas-liquid reactions, stirred tank or fluidized bed reactors are preferred.
Physicochemical properties: Factors such as viscosity, density and reactivity determine whether a reactor with high agitation or high pressure is used.
Reaction speed and selectivity: Fast reactions may require small reactors with high heat and mass transfer efficiency. Processes where high product selectivity is sought, such as alkylation, demand reactors that minimize the formation of unwanted by-products.
Temperature and pressure: Reactors designed for extreme conditions, such as hydrocrackers, must be robust and provide operational safety.
Residence time: Depending on the kinetics of the reaction, a design is chosen that optimizes the contact time between reactants and catalyst.
Catalyst type: The catalyst used directly influences the reactor design. Solid catalysts use fixed or fluidized bed reactors, liquid or gaseous catalysts require reactors with continuous mixing or special configuration to minimize losses.
Cost and economic efficiency: Reactors must balance installation, maintenance and operating costs. Complex processes may justify higher investment reactors if they generate higher yields or reduce operating costs.
Safety and environmental requirements: Reactors for hazardous processes (such as alkylation) must be designed with advanced safety systems. Environmental factors, such as the generation of by-products or emissions, also influence the selection.
Process scale: In large-scale refineries, modular or optimized design reactors are preferred for ease of maintenance and future expansion.
Role of reactors in the hydrocarbon refining process
Reactors play an essential role in improving the quality of refined products by transforming crude oil and its fractions into more valuable and specific products. This is achieved through controlled chemical reactions, optimized by reactor design and operating conditions. The mechanisms by which reactors contribute to improving the quality of refined products are detailed below:
Elimination of impurities: Catalytic reactors eliminate undesirable compounds such as sulfur and heavy metals that affect fuel performance, producing products with lower pollutant emissions and better engine performance.
Increased octane rating: Reforming reactors produce aromatic compounds that raise the octane rating of gasoline, improving its efficiency and quality.
Molecular weight reduction: Cracking in reactors converts heavy fractions into lighter and more useful fuels such as gasoline and diesel.
Optimization of specific gravity and viscosity: Reactors performing hydrotreating and hydrocracking reactions modify the physical properties of refined products, reducing the viscosity of heavy fractions and adjusting the specific gravity of fuels to ensure compatibility with engine standards.
Generation of specific products: The design and operating conditions of the reactor allow obtaining low sulfur products for marine and land applications, high octane gasoline by alkylation and reforming, and high purity lubricants, improving thermal and chemical stability.
Thermal reaction control and selectivity: The reactors are designed to control temperature and pressure, optimizing endothermic reactions (e.g. catalytic reforming) that produce high value products without thermal degradation and exothermic reactions (e.g. alkylation) that control heat release to avoid the formation of unwanted by-products.
Catalyst utilization: The reactors enhance the action of catalysts, which are essential to increase the reaction rate, improve the efficiency of the process and improve selectivity towards desired products, minimizing the formation of impurities and residues.
Cleaner and more efficient fuel production: The ability of reactors to optimize fuel chemistry reduces their environmental impact and improves the performance of diesel and gasoline.
Flexibility to process crudes of different quality: In modern refineries, reactors allow the processing of heavy crudes or crudes with high levels of impurities:
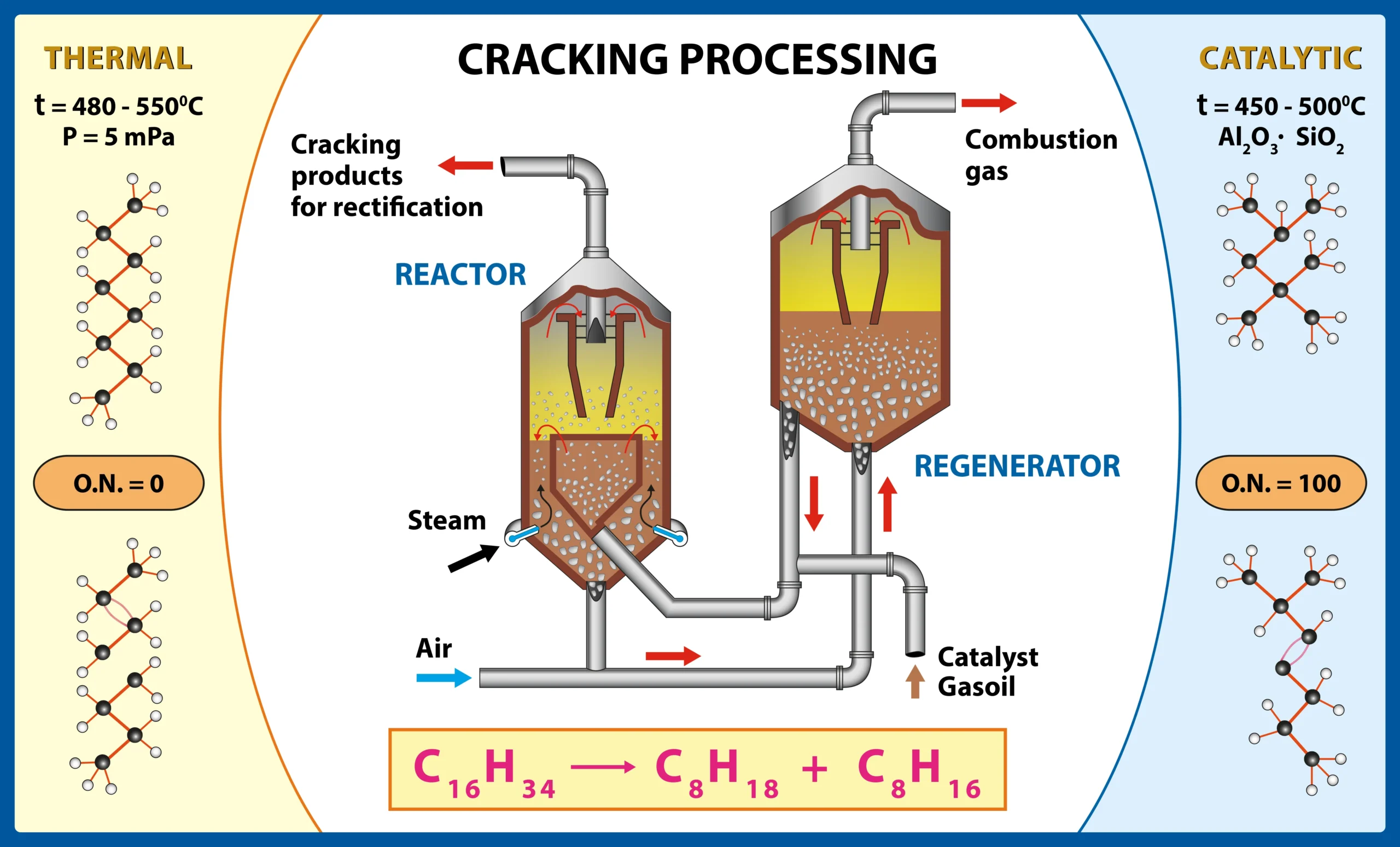
Challenges and trends in reactors design
With technological advances, reactors must adapt to the demands of sustainability and efficiency. Current designs focus on:
- Improve heat and mass transfer.
- Increase the useful life of catalysts.
- Reduce emissions associated with refining processes.
One example of innovation is the use of computational simulations to optimize reactor design and operation, which makes it possible to anticipate problems and maximize performance.
Conclusion
Reactors play a crucial role in hydrocarbon refining, determining the quality and quantity of the products obtained. The selection of the right type of reactor and catalyst is essential to the success of industrial processes such as catalytic cracking and hydrotreating. As the industry evolves, advances in design and technology will continue to transform the refining process landscape, enabling more efficient, safe and sustainable operation.
References
- https://www.chemengghelp.com/fixed-bed-reactor/
- https://es.slideshare.net/slideshow/fluidized-cataltic-cracking/111603643
- https://www.researchgate.net/figure/Alkylation-reactor-module_fig2_359178152
- https://www.biorender.com/template/stirred-tank-reactor-design.
- https://www.shutterstock.com/es/image-vector/producing-fuel-crude-oil-catalytic-cracker-1311074066