Introduction
Catalysis plays a fundamental role in the chemical industry, facilitating the acceleration of reactions through the reduction of the activation energy, without the catalyst undergoing permanent modifications. Its implementation allows to optimize conversion efficiency, decrease energy consumption and reduce the generation of unwanted by-products. It contributes to the viability of multiple industrial processes, from hydrocarbon refining to the synthesis of high value-added compounds, such as pharmaceuticals and specialty polymers.
Recent developments in catalyst design have improved the activity, selectivity, and stability of catalytic systems, enabling higher substrate conversion and minimizing deactivation by poisoning or sintering. Advanced technologies, such as photocatalysis and enzymatic catalysis, have expanded the applications of these materials in processes that seek to maximize chemical efficiency and reduce environmental impact through the use of more sustainable synthetic pathways.
This article examines recent advances in catalysis, their applications in industry and their influence on chemical process optimization from an energy efficiency and sustainability perspective.
Chemical catalysis
Catalysis consists of accelerating a chemical reaction through the presence of a catalyst, which is a substance that is not consumed and facilitates the formation of products. Its use optimizes the efficiency of multiple industrial processes, reducing energy consumption and improving selectivity in the production of chemical compounds.
Types of catalysis and their industrial applications
Depending on the phase in which the reactants, products, and catalysts are found, catalysis is classified into different types, each with specific characteristics that determine its applicability in different industrial processes.
Homogeneous catalysis
In homogeneous catalysis, reactants, products, and catalyst coexist in the same phase, generally in solution. This type of catalysis allows a high interaction between the reactants and the catalyst, which facilitates precise control of the reaction and improves selectivity, increasing chemical reaction rates. However, the separation of the catalyst from the reaction medium is more complex, which can increase operating costs. The most commonly used homogeneous catalysts include organometallic complexes, used in processes such as hydroformylation and selective oxidation.
Their application is common in polymer production and in the conversion of organic compounds.
Heterogeneous catalysis
Heterogeneous catalysis occurs when the catalyst is in a different phase than the reactants and products, such as in solid-liquid or solid-gas systems. The reaction takes place at the surface of the catalyst, where active centers facilitate chemical conversion. This type of catalysis is widely used in industry due to its stability and reusability, reducing operating costs and minimizing waste. An example is oil refining, where solid transition metal catalysts favor fuel desulfurization.
It is widely used in the petrochemical industry, in the synthesis of chemical products and in the production of biofuels.
Heterogenized catalysis
This type of catalysis combines features of homogeneous and heterogeneous catalysis. In these systems, the catalyst can generate soluble intermediates that participate in homogeneous cycles before regenerating into their original heterogeneous phase. This allows improving the catalytic activity and facilitating the separation of the catalyst, achieving a balance between efficiency and ease of recovery. Its application is relevant in processes such as hydrodesulfurization and the production of fine chemicals.
Enzymatic catalysis
Enzymatic catalysis employs enzymes as biological catalysts of high specificity and efficiency. These macromolecules, generally of a protein nature, facilitate reactions under mild conditions of temperature and pressure, minimizing the use of aggressive reagents. Enzyme catalysis is applied in processes such as hydrolysis, ring-opening polymerization and condensation reactions. In addition, immobilized enzymes can be recovered and reused, increasing the sustainability of the processes.
It is used in biochemical reactions, in the pharmaceutical and food industries. Its efficiency and specificity have boosted its use in biotechnology and sustainable synthesis processes.
Recent advances in catalysis
In recent years, the development of new catalysts has improved the efficiency and selectivity of multiple chemical processes. The implementation of advanced characterization and modeling techniques has facilitated the creation of more resistant, stable and reaction-specific catalysts.
Advanced molecular design
The design of catalysts with optimized structures at the molecular level has revolutionized the efficiency of key reactions in industry. Through materials engineering and nanotechnology, catalysts with more accessible active sites and greater stability have been developed, allowing improved performance in complex chemical processes.
Hydrogen economy and catalysis
Catalysis plays an essential role in the production and storage of hydrogen, considered a key energy vector in the transition to renewable sources. The optimization of catalysts for water electrolysis and hydrocarbon reforming has improved the efficiency of these processes, reducing costs and increasing the viability of hydrogen as a clean fuel.
Sustainable chemical reactions
Catalysis has enabled the development of cleaner and more efficient processes, aligned with the principles of green chemistry. Reducing the use of toxic solvents, minimizing waste and taking advantage of renewable resources are some benefits of new catalytic technologies.
Recent advances in catalysts
The development of more efficient and sustainable catalysts has boosted the optimization of chemical processes, reducing energy consumption and waste generation. Nanotechnology has played a crucial role in improving catalytic activity, allowing structural manipulation at the atomic and molecular level to optimize the selectivity and stability of catalysts in various industrial reactions.
This video explains three key characteristics of a catalyst to improve the efficiency of the catalytic process: Catalytic activity, Selectivity and Lifetime (stability). Source: Minstrong Company.
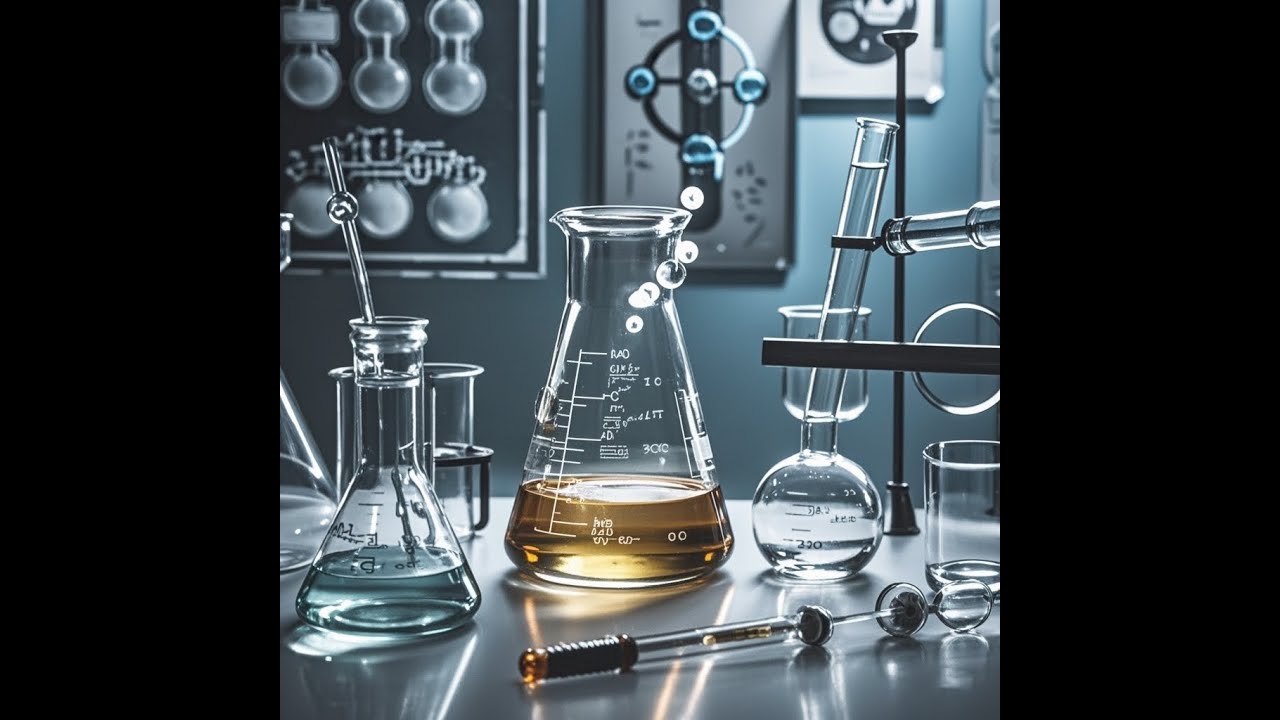
Three main characteristics of catalysts.
Catalysts to produce green hydrogen
Green hydrogen, obtained by electrolysis of water with renewable energy, requires highly efficient catalysts to reduce the overpotential of the hydrogen evolution reaction (HER). Traditionally, iridium has been used in the electrolysis of water in acidic media, but its scarcity and high cost have prompted the search for alternatives. New catalysts based on metal oxides and structures doped with abundant metals, such as iron and cobalt, have been developed, which exhibit high catalytic activity and stability in demanding operating media.
A new electrocatalyst is capable of producing green hydrogen from water. The invention makes it possible to produce clean hydrogen on an industrial scale. A catalyst that accelerates this chemical reaction is used to break down water molecules (H₂O) and extract hydrogen (H2). At present, the researchers comment, catalysts made of materials such as platinum are used, which, in addition to being expensive and hard to find, are not very efficient.
In a study published in the journal ‘Nano Energy’, they explain how using nickel and cobalt in one of these catalysts they have managed to break the water atom molecules using less energy and achieving greater hydrogen extraction. The following image shows the electrochemical arrangement of this process.
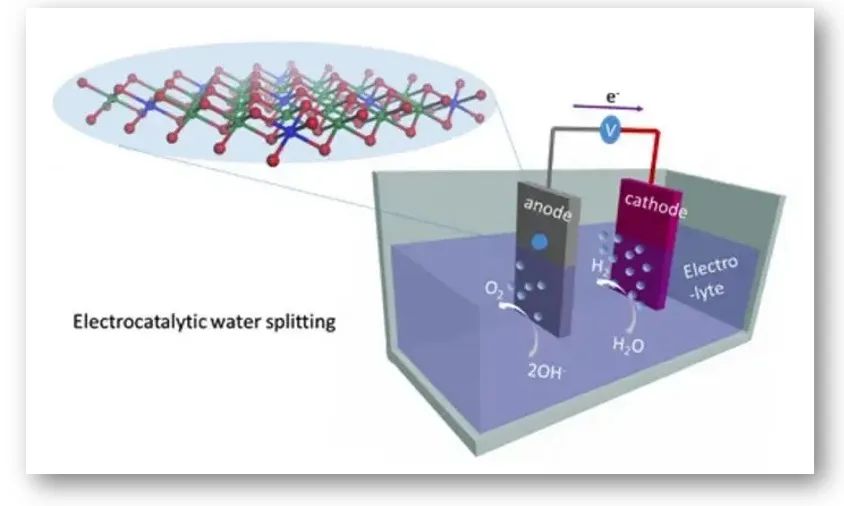
Liquid metal-based catalysts
Liquid metals, such as gallium, have demonstrated unique properties in heterogeneous catalysis, as they can dissolve elements such as nickel and tin, generating catalytic systems with high activity at reduced temperatures. This type of catalysts has shown efficiency in the activation of C-H bonds and in the conversion of organic compounds through mechanisms that minimize the formation of undesired by-products. Their stability and self-regeneration capacity make them a promising alternative for industrial processes with low energy consumption.
Tungsten oxide-based catalysts.
Tungsten oxide (WO₃) has proven to be a versatile catalytic material, with applications in photocatalysis, electrochemical CO₂ reduction and sustainable chemical synthesis. Its ability to act as a semiconductor in photoinduced processes allows its use in the degradation of organic pollutants and in the production of solar fuels. In addition, its thermal stability and resistance to deactivation make it a candidate for large-scale applications in the chemical industry.
Single-atom catalysts
Single atom catalysts (SACs) represent a new frontier in heterogeneous catalysis, where active metal atoms are isolated and anchored on specific supports, maximizing their catalytic efficiency. These systems have shown superior performance in the conversion of feedstocks into synthetic fuels and in the selective reduction of nitrogen oxides (NOₓ). Their structural design allows for improved activity, minimized metal waste and increased stability in long-lasting reactions.
Nickel-based catalysts
Nickel has gained relevance in catalysis due to its abundance and activity in hydrogenation reactions, hydrocarbon reforming and bioremediation processes. It has been demonstrated that nickel-based catalysts can be reusable without significant loss of activity, which makes them suitable for the removal of organic pollutants in aqueous media. In addition, their interaction with functionalized supports improves their selectivity and resistance to deactivation by poisoning.
Applications of advances in catalysis
Recent developments in catalysis have broadened their impact in a variety of industries, including:
- Biofuel production: Optimization of processes for the conversion of biomass into renewable fuels with a lower carbon footprint.
- Artificial photosynthesis: Use of advanced catalysts for the conversion of sunlight into chemical energy through water splitting and CO₂ fixation.
- Pollution reduction: Application of catalysts in the removal of persistent organic compounds in water and in the capture and conversion of gaseous pollutants.
- Synthesis of high commercial value products: Development of more efficient catalytic routes for the production of pharmaceuticals, polymers, and specialty chemicals.
These advances continue to transform industrial catalysis, promoting more sustainable and efficient processes in global chemical production.
Importance of catalysis in the chemical industry
Catalysis is fundamental in the chemical industry, as it allows reactions to be carried out with greater selectivity and speed in a more efficient manner and with less energy expenditure. Its impact can be seen in the synthesis of key products such as fuels, plastics, fertilizers and pharmaceuticals. It also contributes significantly to the reduction of polluting emissions and the development of more sustainable processes, in line with green chemistry.
One of the major benefits of catalysis is its contribution to industrial sustainability. By reducing the energy required for chemical reactions, it reduces greenhouse gas emissions and minimizes waste generation. This aligns with the principles of green chemistry, promoting the use of more selective and less polluting catalysts.
In sectors such as petroleum refining, hydrogen production and the synthesis of pharmaceuticals, advances in catalysis have enabled the development of more efficient and environmentally friendly processes. Moreover, the use of innovative catalysts, such as those based on nanotechnology or enzymes, opens up new possibilities for improving the economics and sustainability of the chemical industry in the future.
Conclusions
The choice of the type of catalysis depends on factors such as the nature of the reagents, the required selectivity and the economic feasibility of the process. While homogeneous catalysis offers high reactivity, heterogeneous catalysis stands out for its ease of recovery. Heterogeneous catalysis represents a hybrid approach with combined advantages, and enzymatic catalysis enables the development of more sustainable biotechnological processes. Optimization of these systems remains a key area of research and development in the chemical industry.
Recent developments in catalysis, such as nanometer, single atom and enzymatic catalysts, have improved process selectivity and efficiency. These innovations contribute to the transition to more sustainable processes, aligned with the principles of green chemistry. Catalysis plays a key role in mitigating the environmental impact of the chemical industry. Its use makes it possible to reduce energy consumption, reduce waste generation and limit pollutant gas emissions, favoring a more sustainable development.
References
- Guohua Jia; “Activating basal planes of two-dimensional iron sulfide nanosheets for efficient hydrogen evolution reaction”; Nano Energy, septiembre de 2021.
- Somorjai, G. A., & Li, Y. (2010). Introduction to Surface Chemistry and Catalysis. John Wiley & Sons.
- Ertl, G., Knözinger, H., & Weitkamp, J. (Eds.). (2008). Handbook of Heterogeneous Catalysis. Wiley-VCH.