Introduction
Materials science research is critical to developing technologies that promote a sustainable energy future. By designing and producing advanced materials and environmentally friendly manufacturing processes, the implementation of cleaner and more efficient energy systems is facilitated. Sustainable materials innovations that impact energy efficiency optimize energy generation, storage and distribution, and also contribute to reducing pollutant emissions.
The integration of sustainable practices in this field fosters collaboration between various scientific and technological disciplines, driving the development of future energy systems that are both innovative and environmentally friendly.
What is materials science?
Materials science is an interdisciplinary scientific discipline that studies the relationships between the internal structure of materials and their macroscopic properties. It analyzes how atomic and molecular arrangement influences physical, chemical, mechanical, electrical, magnetic and optical characteristics. This knowledge makes it possible to design and manufacture materials with specific properties for applications in fields such as physics, chemistry, metallurgy, ceramics, polymers and engineering.
Materials engineering is based on these principles to design structures that achieve predetermined properties. In addition, this science is the basis for advances in nanotechnology, quantum computing, energy storage and other technologies. More recently, artificial intelligence has been driving the development of new materials and advanced manufacturing processes.
The nexus between materials science and renewable energies
The efficiency of renewable energies is intrinsically linked to the materials used in the capture, conversion, storage and distribution of energy. Materials science focuses on studying the microstructure, physicochemical properties, processing methods and functional performance of these materials, allowing the design and synthesis of new composites with specific characteristics that optimize the performance of renewable technologies.
Unlike fossil fuels, which generate energy through combustion, renewable sources harness natural phenomena such as sunlight, wind, water or geothermal heat. In this context, innovation in sustainable materials is strategic to address global energy challenges. The constant search for cleaner, low-carbon and more efficient solutions is closely linked to the development of innovative materials that offer greater durability, storage capacity and sustainability, using renewable raw materials.
Materials science for sustainability focuses on a few core research areas
- Clean energy conversion, storage and distribution: Development of advanced materials that improve the efficiency of devices such as solar cells, batteries and energy storage systems.
- Circular materials that replace rare, high-energy or hazardous materials: Research into sustainable alternatives that reduce dependence on scarce or harmful materials, promoting the circular economy.
- Mitigation, cleanup and protection of the atmosphere, soil and water: Design of materials that contribute to the decontamination and preservation of the environment, such as catalysts for air and water purification.
- Materials discovery for new sustainable technologies: Exploration of innovative compounds that drive the development of emerging technologies with low environmental impact.
Specific examples of these advances include
- Fully recyclable batteries: Design of energy storage systems that facilitate their complete recycling at the end of their useful life.
- Future fuels with minimal carbon footprint: Development of sustainable fuels that reduce greenhouse gas emissions.
- Air, water and soil cleaning through solar energy: Use of photocatalytic materials that, activated by sunlight, degrade environmental pollutants.
- Energy-efficient electronics for the digital society of the future: Creating electronic components that consume less energy, contributing to sustainability in the digital age.
- Materials for tomorrow’s sustainable technologies and infrastructure: Innovation in building materials and other sectors that promote sustainable and resilient practices.
- Integrating these approaches into materials science is necessary to develop future energy systems that are innovative and ecologically responsible.
The following video shows how researchers at the Complutense University of Madrid have developed an innovative solar cell that could achieve an energy conversion efficiency of 60%. Using gallium phosphide (GaP) doped with titanium (Ti), they have created an intermediate band structure that exceeds the theoretical efficiency limit of traditional solar cells. This breakthrough promises to revolutionize solar power generation, offering a more efficient and sustainable source.
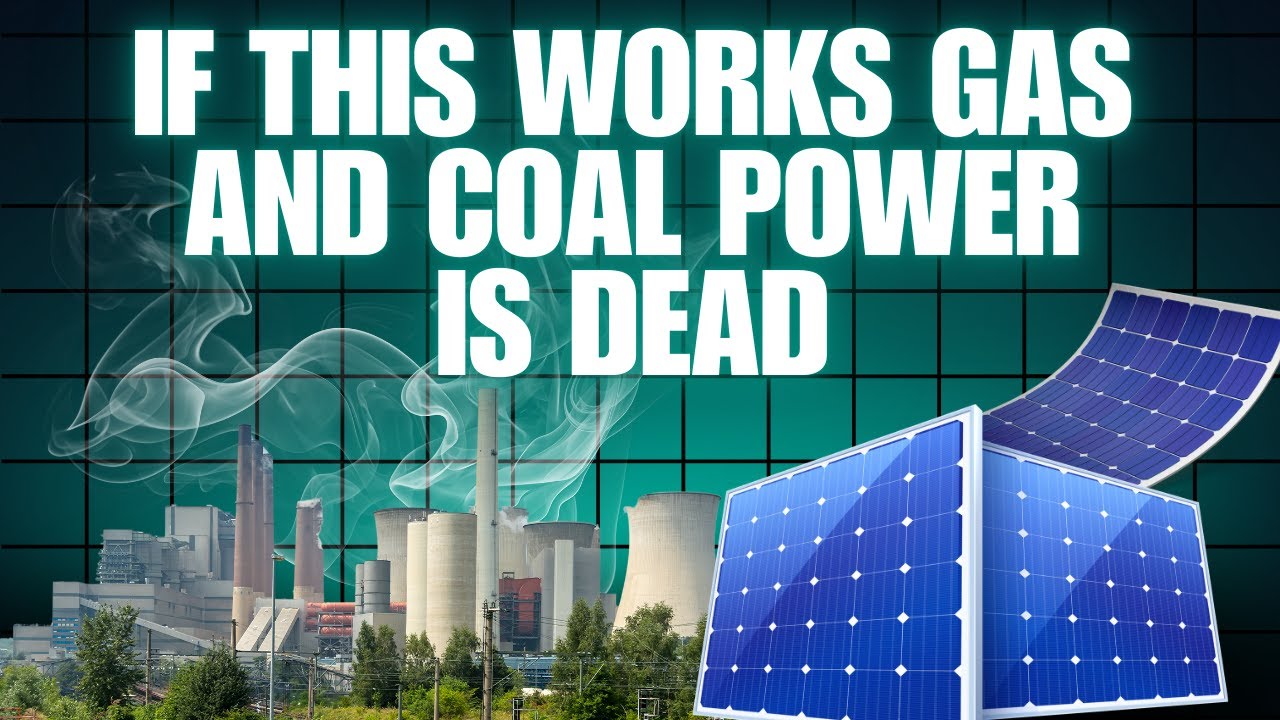
Advanced raw materials for a sustainable energy future
Available raw materials are the basis for the development of renewable energy technologies. The availability and access to materials such as lithium, cobalt, nickel and rare earths are essential for the production of these technologies. However, it is necessary to consider the environmental and social impact of the extraction of these materials.
Research into advanced low-carbon materials is ongoing, seeking more sustainable alternatives that are abundant, recyclable, biodegradable and have a lower environmental impact in their life cycle.
Contributions of materials science to renewable energies
Materials science is the basis for the development of innovative technologies that harness energy from the sun, wind, water and other renewable sources more efficiently and cost-effectively. Its contributions are manifested in several areas:
Development of advanced materials
The creation of materials with optimized properties, such as high energy efficiency, electrical conductivity and mechanical strength, is necessary to improve the performance of equipment such as solar panels, wind turbines and storage batteries.
For example: In solar panels, anti-glare and self-cleaning coatings are being investigated, along with high-efficiency, low-cost perovskite cells. Wind turbines take advantage of lightweight, strong composite materials, such as carbon fiber, for larger, more efficient blades. Storage batteries explore graphene and silicon electrodes for higher capacity and faster charging, as well as new chemistries such as lithium-sulfur and sodium-ion, which are more economical and sustainable.
Innovation in sustainable materials
The search for materials that are functional and environmentally friendly involves the development of renewable, recyclable, biodegradable and durable options that minimize environmental impact at all stages of the life cycle, from raw material extraction to manufacture, use and disposal. For example, biodegradable materials are being investigated for wind turbine and solar panel components, as well as recyclable materials for batteries and other devices.
Ease of recycling and reuse
The development of new materials drives more effective recycling technologies, reducing waste and reducing the need to extract new resources. For example, in the manufacture of solar panels, the implementation of circular economy practices makes it possible to recover and reuse components at the end of their useful life, reducing the environmental impact and associated costs. Innovative processes have been developed to separate and recover the valuable materials that make up solar panels, such as silicon, silver and aluminum.
Promotion of new technologies
This discipline promotes innovations such as solid-state batteries, next-generation energy storage systems, thermoelectric materials and optoelectronic devices, which are strategic for the integration of renewable energies into the power grid and the development of new energy applications. For example, solid-state batteries promise to be safer and more efficient than conventional lithium-ion batteries, while thermoelectric materials could be used to convert waste heat into electricity more efficiently.
Interdisciplinary
Materials science is characterized by its interdisciplinary approach, integrating knowledge and methods from disciplines such as chemistry, physics, engineering and nanotechnology. These collaborations develop solutions in the field of materials, achieving a comprehensive understanding and enhancing scientific and technological advances.
Energy efficiency as a priority objective
Energy efficiency is a key factor for the development and consolidation of renewable energies. Materials science contributes to this objective through the development of innovative materials that enable:
Minimize energy losses: Optimize energy generation, storage and distribution by using materials with low electrical resistivity, high thermal conductivity and low energy dissipation, improving efficiency in electrical and thermal systems. For example, superconducting materials are being developed that could transport electricity without losses, which would revolutionize long-distance energy transmission.
Optimizing device performance: Improving efficiency in solar panels, wind turbines and batteries through advanced materials with specific properties for greater energy conversion and storage. For example, anti-reflective coatings are being used on solar panels to increase the amount of light they capture, and new battery electrode materials are being developed to store more energy.
Reducing energy consumption: Application of ultralight, insulating and reflective materials in buildings, transportation and industry, reducing energy demand for heating, cooling and mobility. For example, high-efficiency insulating materials are being used in buildings to reduce the need for heating and cooling, and lighter vehicles are being developed with composite materials to reduce fuel consumption.
The development of materials with properties optimized for energy efficiency is required to achieve a successful and sustainable energy transition, enabling a more rational and efficient use of available energy resources.
Factors to consider
- Scalability of production: Many promising materials are in the research or development phase on a small scale, and methods need to be found to produce them on a large scale in an efficient and cost-effective manner.
- Durability of materials: Some materials used in renewable energy devices may degrade over time due to exposure to weathering, corrosion or other factors, reducing their useful life and efficiency.
- Cost reduction: The cost of some renewable energy materials and technologies remains high, making mass adoption difficult.
Conclusion
Materials science is central to the transition to renewable energy, driving the development of more efficient and sustainable technologies. Research into new materials optimizes the generation, storage and distribution of clean energy, addressing technological and environmental challenges. Collaboration between scientists, engineers and industry is key to accelerating this transition and building a sustainable energy future.
References
- https://kaw.wallenberg.org/en/materials-science-sustainable-world#:~:text=The%20Wallenberg%20Initiative%20Material%20Science%20for%20Sustainability%20focuses%20on%20four,and%20water%20and%20discovery%20of
- https://www.elsevier.es/en-revista-ciencia-tecnologia-dos-materiais-226-articulo-research-on-materials-renewable-energy-S0870831217300071