Since ancient times, metals and their alloys have represented a fundamental alternative solution in technological development to solve humanity’s greatest challenges. The constant search for materials with optimized properties led to the discovery of high entropy alloys (HEA) in 2004. These represent a paradigm shift in materials science, introducing a group of alloys characterized by their equimolar composition of five or more major elements.
Their unique crystalline structure and high mixing entropy open up new possibilities in the design of materials with exceptional properties, broadening the horizons of research and industrial applications. In addition to their mechanical properties, they exhibit innovative functional characteristics, such as superparamagnetism, soft magnetic properties and superconductivity. These qualities broaden their application in areas such as superconducting motors and generators, where the type-II superconductors of these alloys stand out for their mechanical hardness and conventional behavior in superconducting waves.
This article aims to provide a comprehensive overview of high entropy alloys (HEAs), from their definition and main characteristics to the technological advances in their synthesis. The current applications of these alloys in strategic sectors such as energy, aerospace and health will be analyzed, highlighting their ability to overcome the limits of conventional alloys. In addition, the future impact of HEAs in materials engineering will be explored, considering the challenges and opportunities they pose in the development of sustainable and innovative solutions.
What are high entropy alloys and what do they consist of?
High entropy alloys are an innovation in materials science, characterized by mixing equal or relatively large proportions of at least five major elements, configured in highly symmetric crystal structures such as face-centered cubic (FCC), body-centered cubic (BCC) or hexagonal compact (HCP).
They are characterized by their multicomponent mixture of elements, exhibit excellent properties, such as high thermal stability, corrosion and wear resistance, and remarkable hardness. For example, HEAs with BCC structure (TaNbMoW, TaNbMoW, TaNbHfZrTi) are distinguished by their thermal stability and hardness1, while those with FCC phase (CrMnFeCoNi, AlCrMnFeCoNi) offer better ductility2.
This approach contrasts with traditional alloys, which are usually based on one or two dominant components, supplemented with small amounts of other elements to improve specific properties. For example, conventional steels are based on iron with relatively low proportions of carbon, manganese or other additives.
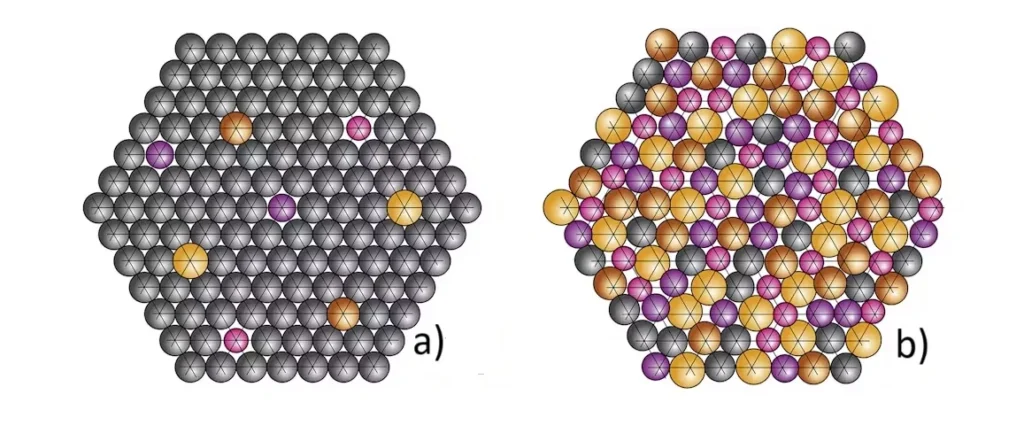
The term “high entropy alloys” was introduced by Taiwanese scientist Jien-Wei Yeh. His definition highlights the significant increase in mixing entropy when multiple elements are combined in similar proportions. This increase in entropy favors the thermal and mechanical stability of the phases formed, making these alloys exhibit more homogeneous and resistant crystalline structures.
In addition to this name, some researchers have proposed alternative terms such as “multicomponent alloys”, “complex composition alloys” or “multi-main element alloys”, reflecting their diverse and complex nature.
HEAs have captured attention in engineering and materials science because of their ability to offer desirable mechanical and functional properties. Among their most outstanding characteristics are their high corrosion resistance, toughness, thermal stability and, in some cases, an excellent combination of strength and ductility. These properties position them as ideal materials for applications in critical sectors such as aerospace, energy and military, where conventional materials could fail under extreme conditions.
Despite their great potential, HEAs also face significant challenges. These include the high costs associated with their synthesis, complexity in controlling their microstructures and scalability for industrial production. However, advances in materials processing and characterization technology, coupled with a multidisciplinary approach to their research, are paving the way to overcome these obstacles.
Evolution of supermaterials or high entropy alloys
The following is a historical overview of the progress of metal alloys over time:
Breaking the limits of traditional alloys
For millennia, advances in metallurgy seemed to reach an insurmountable limit: adding too many elements to an alloy resulted in negative properties, such as brittleness. However, in 2004, researchers Brian Cantor and Jien-Wei Yeh transformed this view. They discovered that, by increasing the entropy of a metallic mixture above a critical threshold, it was possible to obtain single-single-phase alloys, where the atoms are randomly distributed. Thus, high entropy alloys (HEA) were born, revolutionizing metallurgy and opening a new horizon in materials science.
From traditional rules to new frontiers
The initial HEA concept focused on equiatomic mixtures, but was soon extended to more flexible combinations. These alloys have proven to be competitive in key properties, such as high temperature resistance, hydrogen storage capacity and magnetic applications. In less than two decades, scientific publications on this type of material have grown from a few to thousands, setting an accelerating pace in the field.
A new era of materials?
HEAs represent a paradigm shift comparable to milestones such as the discovery of steel or bronze. As we search for solutions to current technological limits, these alloys offer a gateway to significant breakthroughs in different industrial sectors. High entropy alloys promise such improvements, and are attracting increasing research interest due to this unique combination of structural randomness and excellent properties, opening up new possibilities in advanced materials design.
The synthesis of high entropy alloys: methods and technological advances
High entropy alloys (HEA) present significant challenges in their manufacture due to the complexity of their composition and the limitations of available techniques. These alloys, which combine multiple elements in equimolar or close proportions, require specialized methods to achieve homogeneous structures and optimized properties. The main approaches in their synthesis are described below, highlighting the advances and limitations of each technique.
Metal phase-based methods
- Liquid phase processing: This method includes techniques such as arc melting, induction melting and Bridgman solidification, widely used for HEA production. Melting allows metals to be combined in a liquid state, favoring the formation of alloys with controlled structures. However, rapid cooling can generate unbalanced microstructures, which requires precise adjustments to the process parameters.
- Solid-state processing: Mechanical alloying, using high-energy ball mills, is the main technique in this approach. This method produces metal powders that can be transformed into functional parts by powder metallurgy or spark plasma sintering. This process is especially useful for manufacturing alloys that are difficult to produce by casting, such as LiMgAlScTi. Additionally, the resulting powders can be spheroidized for use in additive manufacturing, improving their flowability and uniformity. A notable advance is the sequential alloying method, proposed by Vaidya et al. that allows greater flexibility in the formation of crystalline phases (BCC and FCC). This method consists of adding elements step by step, which can significantly influence the final properties of the alloy.
- Gas phase processing: Methods such as sputtering and molecular beam epitaxy (MBE) allow the fabrication of thin films with controlled elemental compositions. This approach is ideal for developing high entropy metallic or ceramic coatings with specific properties.
- Additive manufacturing has emerged as a key tool in HEA synthesis, as it allows obtaining unique microstructures that combine high strength (up to 1.3 GPa) with improved ductility. Complementary techniques such as thermal sputtering, laser cladding and electroplating extend the design possibilities of these alloys, adapting them to various industrial applications.
High entropy alloys and their impact on materials science
Advances in the properties and design of stable crystalline structures
High entropy alloys (HEAs) represent an innovative class of metallic materials, characterised by high configurational entropy, derived from the random arrangement of multiple elements in a crystal lattice, resulting in a complex microstructure with appropriate mechanical properties such as high strength, ductility, toughness and corrosion resistance.
The uniqueness of HEAs is not limited to their mechanical properties; they also stand out for their thermal stability and wear resistance, making them ideal for applications in industries such as aerospace, automotive and energy. This phenomenon, regulated by the Gibbs free energy equation (ΔGmix = ΔHmix – TΔSmix), allows the stabilisation of single crystal structures, such as face-centred cubic (FCC), body-centred cubic (BCC) and compact hexagonal (HCP), in the context of materials science.
Impact on the problem of hydrogen corrosion in materials
High entropy alloys not only represent a breakthrough in materials design, but are also redefining the understanding of critical phenomena in materials science. A key example is their potential to solve the problem of hydrogen embrittlement.
Traditional austenitic steels, commonly used in hydrogen vessels, exhibit embrittlement when interacting with hydrogen, limiting their reliability and safety. Faced with this problem, high entropy alloys (HEAs) emerge as an alternative solution due to their advanced properties.
HEAs, composed of more than five elements in similar proportions, have complex crystal structures that increase their resistance to hydrogen embrittlement. Researchers such as those at the Polytechnic University of Madrid (UPM) are using advanced simulations to understand how lattice configurations and modifications to the morphology of the internal phases of these alloys can minimise the diffusion of hydrogen atoms. This approach aims to develop safer and more efficient materials for hydrogen-related applications.
In addition, HEAs offer significant advantages over traditional metals, such as higher mechanical strength, improved ductility and higher temperature stability. These characteristics make these alloys a viable alternative for hydrogen storage vessels and transport systems under aggressive conditions.
High entropy alloys (HEAs) applications
High entropy alloys have revolutionized multiple industrial sectors due to their unique composition and exceptional properties. Their main applications are described below:
1. Energy storage
Advanced materials play a key role in improving the capacity and extending the life cycle of energy storage devices. High entropy materials (HEMs) with controlled compositions and simple phase structures have attracted the interest of researchers and have undergone rapid development recently.
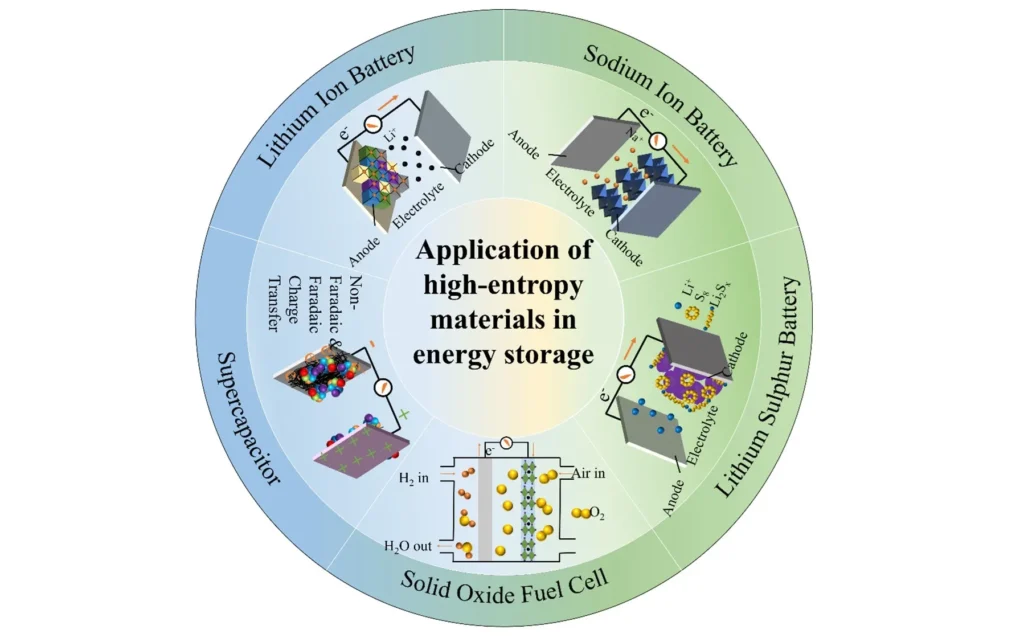
HEAs play a crucial role in batteries, fuel cells and supercapacitors, improving capacity, lifetime and electrochemical performance. Their high-entropy structures facilitate the stability and efficiency of electrode materials, opening up new possibilities in advanced energy storage devices.
2. Catalytic applications for clean energy conversion
HEAs have proven to be highly active and durable catalysts in key reactions such as:
- Hydrogen evolution.
- Oxygen evolution.
- Oxygen reduction.
- Carbon dioxide reduction (CO₂).
- Ammonia decomposition (NH₃).
Due to their selectivity and stability, they drive clean and sustainable energy technologies.
3. Aeronautics and aerospace technology applications
Because of their low weight, high strength and ability to withstand extreme temperatures, HEAs are essential in aircraft and rocket structural components; these properties also make them ideal for highly corrosive environments, and they have uses in critical satellite and space vehicle applications. They represent key materials for coping with the extreme conditions of space.
4. Thermoelectric (TE) applications
The advancement of thermoelectric technology (TE) is increasing rapidly as the demand for energy is constantly increasing. On the one hand, a large amount of renewable energy is used to meet the growing energy demand. On the other, TE technology is an attractive option as it can utilize waste heat energy. In addition, TE materials have a small operating size and can be applied to solid-state cooling, portable and wearable electronics, etc.
In TE technology, different advanced materials are used to convert waste heat into electricity. The most common thermoelectric materials are Zintl, Heusler, MgSi, among others. However, several high entropy alloys are emerging as new TE materials of interest with high temperature properties.
5. Oil & Gas Industry
HEAs offer superior resistance to corrosion, wear and high temperatures, making them ideal for pipelines, valves and extraction equipment in severe conditions. High entropy alloys (HEA) are revolutionizing the Oil & Gas industry due to their ability to withstand extreme operating conditions. These alloys, characterized by a balanced chemical composition of multiple elements, offer a unique combination of properties such as high corrosion resistance, thermal stability and exceptional mechanical durability.
In the Oil & Gas industry, these characteristics are crucial for components exposed to aggressive environments, such as subsea pipelines, high-pressure valves and equipment used in the extraction and transportation of hydrocarbons. Their wear resistance and superior performance in extreme temperatures make them ideal for improving reliability in both deepwater and acidic environments.
In addition, these materials are key in the development of new technologies, such as protective coatings and more efficient drilling tools, aligning with the industry’s need to reduce costs and increase sustainability in critical processes.
6. Biomedical applications
An additional technological application of interest for high entropy alloys (HEA) is their use in advanced biomedical components. Thanks to their excellent corrosion resistance, high biocompatibility and customizable mechanical properties, they are currently used in the construction of some biomedical components such as:
- Orthopedic and dental implants: HEA can replace traditional materials such as titanium and stainless steel, offering better durability and lower risk of rejection in the human body.
- Surgical devices: Their hardness and wear resistance make them ideal for high precision surgical tools.
- Customized prostheses: HEA can be manufactured by 3D printing to design patient-specific prostheses, optimizing fit and functionality.
This area of research promises to significantly improve the quality and performance of biomedical devices, marking a milestone in healthcare materials engineering.
Future trends of high entropy alloys (HEAs)
Although more than 400 papers have been published on high entropy alloys (HEAs), understanding of this field is still at an early stage. However, recent research has identified key trends that will mark its future development.
Focus on fundamental and basic studies
HEAs represent an opportunity to expand knowledge in materials science and solid state physics. Unlike conventional alloys, composed of one or two major elements, HEAs require exploration of complex phenomena, such as atomic interactions, entropic intermixing, lattice distortion and structural defect energy, reinforcement, strength, wear, corrosion and oxidation, along with their mechanisms and theories. Understanding these aspects is essential for its development, and also opens new avenues for advanced applications.
Research on promising alloy systems
There is a need to study alloy systems that offer improved mechanical, chemical and physical properties. This responds to the ongoing need to develop alloys that not only outperform traditional alloys in performance, but also offer benefits in terms of cost, energy and sustainability.
Exceeding the performance limits of traditional alloys
A key objective in the development of HEAs is to address technological challenges unattainable with conventional materials, such as the creation of superconductors at room temperature or materials with high hardness and resistance to extreme temperatures and aggressive media, representing a viable and safe alternative for applications related to hydrogen storage and transport.
New frontiers: high entropy ceramics
The high entropy concept is also being applied to materials such as nitrides, carbides and oxides in the manufacture of ceramic materials, which have shown promise for applications such as functional coatings and diffusion barriers, further expanding the scope of HEAs.
Development of new industrial applications
The correlation between experimental data and practical applications is essential to maximize the potential of HEAs. This approach will make it possible to tailor these alloys to the specific needs of industries such as aerospace, automotive and energy.
Below is a video summarizing the future of these alloys, courtesy of: AJE Video Bytes.

High-entropy alloys: The future of alloying.
The era of high entropy alloys: turning challenges into opportunities
High entropy alloys (HEAs) represent a promising frontier in materials science, thanks to their ability to combine elements in an almost unlimited way and achieve unique properties. However, the development of these alloys faces significant challenges, such as the availability of critical metals, their high cost and the risks associated with their handling. These obstacles underscore the need to find sustainable and accessible alternatives to advance in this area.
In this context, HEAs open up new possibilities to address global problems. For example, their design could focus on the recycling of metals from electronic waste, offering an innovative solution for the treatment of difficult-to-separate materials. Recent research, such as that carried out by the IMDEA Materials Institute, has shown that it is feasible to develop HEAs from pre-existing alloys, reusing resources and reducing dependence on scarce materials.
In addition, the applications of HEAs in strategic sectors such as aerospace and bio-health show revolutionary potential. Although the transition between the discovery of new materials and their practical use remains slow, advances in simulation and modeling techniques are accelerating this process, bringing us ever closer to concrete solutions.
Are we entering the Age of High Entropy Alloys? Although it is too early to say for sure, their impact on sustainable development and technological innovation suggests that these alloys could define a crucial chapter in human history.
Conclusions
High entropy alloys stand out for their unique crystalline structure and exceptional properties, such as high mechanical strength, thermal stability and corrosion resistance, which position them as key materials for applications in extreme environments and technologically demanding sectors.
The development of advanced synthesis methods, such as arc fusion and physical vapor deposition, has been crucial in optimizing the properties of HEAs. These advances not only improve the quality of the material, but also expand the possibilities for customization according to the specific needs of each industry.
HEAs offer great potential to transform various industrial sectors, but their mass deployment still faces challenges such as the complexity in their large-scale manufacturing and the need for a better understanding of their behavioral mechanisms. Continued research will be key to overcoming these obstacles and maximizing their impact on sustainable, high-performance solutions.
Could entropy be the key to creating the innovative materials of the future?
References
- O. Senkov et al; “Microstructure and room temperature properties of a high-entropy TaNbHfZrTi alloy”; J. Alloy. Compd, (2011).
- J. He et al; “Effects of Al addition on structural evolution and tensile properties of the FeCoNiCrMn high-entropy alloy system”; Acta Mater, (2014).
- Jianping Huang; “Materiales de almacenamiento de energía de alta entropía: síntesis y aplicación”; Journal of Energy Storage, Volumen 66 , 30 de agosto de 2023.