Introduction
Wind turbines have come a long way since their inception, evolving from simple windmills to sophisticated machines capable of harnessing wind energy with remarkable efficiency. The search for high-efficiency wind turbines has been driven by the need to maximize energy production while minimizing costs and environmental impact. They are devices that transform the kinetic energy of the wind into electricity. They operate by capturing the wind with their large blades, which rotate a shaft connected to a generator, producing energy in a clean and renewable way1.
The design of high-performance wind turbines lies in the concept of aerodynamic optimization. Modern turbine blades are carefully designed to capture as much wind energy as possible. They feature aerodynamically shaped cross-sections, similar to aircraft wings, which create a pressure differential as the wind flows over them. This pressure differential generates lift, which causes the blades to rotate.
This article discusses the latest developments in wind turbine technology and their influence on the energy industry and the global fight against climate change.
Innovation in design and technology
A highlight of recent wind turbine developments is the constant innovation in design and technology. Companies and researchers are on a continuous quest to optimize turbine performance, increase efficiency, and reduce costs. A key focus is the redesign of blades, where lighter and more durable materials are used to improve wind energy capture.
In addition to blade innovation, new technologies are being investigated to make the turbines more adaptable to various wind conditions. This includes advanced control systems that automatically adjust blade orientation to maximize wind capture in real-time, as well as storage technologies that allow surplus energy to be conserved for later use.
A key advance in blade design is the use of variable pitch technology. This allows the blades to adjust their angle relative to the airflow, optimizing performance over a wide range of wind speeds. In light breeze conditions, the blades can be angled to capture more energy, while in high wind conditions, they can be adjusted to avoid overloading the generator and ensure safe operation.
The following video shows how wind turbines generate clean electricity from wind power. Source: ENERGY.GOV.
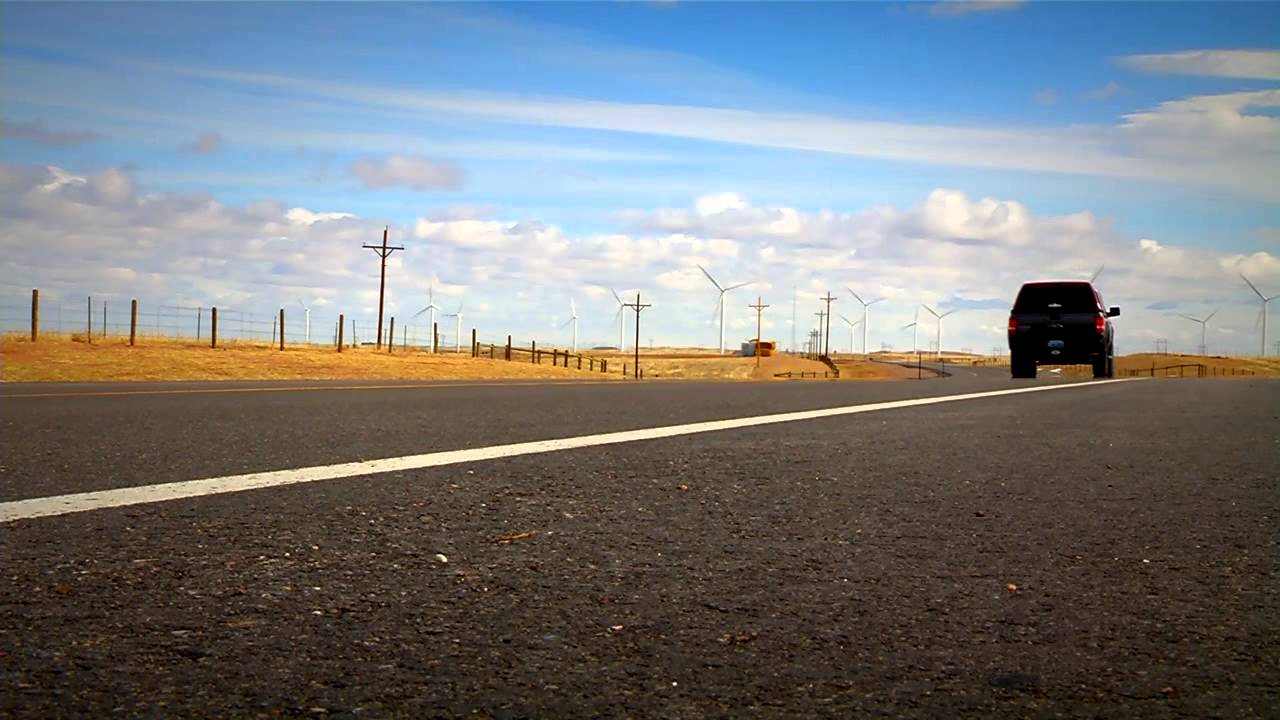
Clean electricity generation from wind energy.
The size of wind turbines has also increased dramatically, contributing to improved efficiency. Larger rotors can capture more wind energy, and taller towers allow turbines to access stronger, more consistent winds at higher altitudes.
Some of the largest turbines now have rotor diameters exceeding 160 meters and can generate up to 5-6 megawatts of power. However, efficiency is not just a matter of size. Advanced materials play a crucial role in the design of high-efficiency turbines. Carbon fiber composites, for example, allow for longer, lighter blades that can capture more energy without adding excessive weight to the structure. These materials also improve durability, reducing maintenance needs and extending the turbine’s operational life.
Advanced materials in turbine components
While blades are a primary focus, advanced materials are also a significant element in wind turbine efficiency:
Superconducting generators: The use of high-temperature superconductors (HTS) in wind turbine generators are materials with no electrical resistance (so they can conduct electricity without losses). They are also promising in the field of power generation: the use of magnets based on superconducting coils in electric generators reduces their weight and dimensions, as well as heating losses.
Figure 1 shows a schematic of a conventional wind turbine, in which the gearbox of the multiplier is much bulkier than the generator. With the new superconducting generator, the multiplier could be less heavy and bulky2.
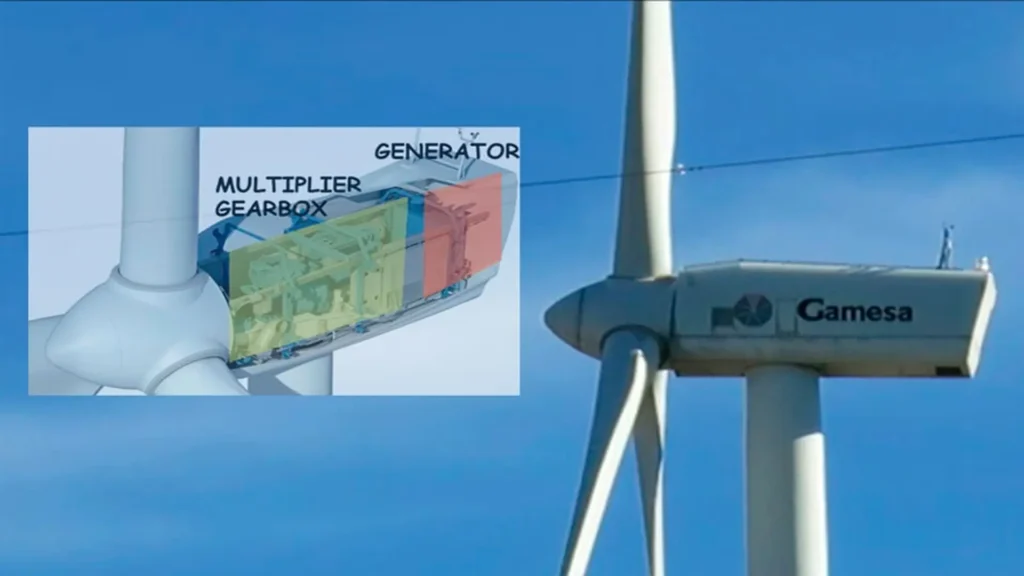
- Rare earth barium copper oxide ribbons (REBCO): These superconducting ribbons can carry more than 100 times more current than copper cables of the same cross-section. A prototype 10 MW HTS generator developed by researchers at the Karlsruhe Institute of Technology demonstrated a 30% reduction in generator weight compared to conventional designs.
- Ultra-high performance concrete (UHPC): this material offers compressive strengths in excess of 150 MPa, enabling taller and slimmer tower designs. A case study published in the Journal of Wind Engineering and Industrial Aerodynamics showed that UHPC towers could increase energy yield by up to 12% for onshore wind farms in low-wind areas.
Benefits of new materials
The development of advanced composite materials has been a key factor in improving the performance and efficiency of wind turbines. These materials offer a combination of high strength, low weight, and durability that is crucial for the ever-increasing size of modern wind turbines.
Carbon Fiber Reinforced Polymers (CFRP)
Carbon fiber composites are at the forefront of wind turbine blade technology due to their exceptional strength-to-weight ratio. Recent advances include:
Researchers have developed hybrid composites that combine the strength of carbon fiber with the cost-effectiveness of fiberglass. A study published in the journal Composite Structures showed that these hybrid composites can increase blade length by up to 25% without a significant increase in weight.
The incorporation of carbon nanotubes into CFRP has shown promising results. A 2023 study in the Journal of Composite Materials reported a 30% increase in interlaminar shear strength when carbon nanotubes were integrated into the epoxy matrix of CFRP.
Thermoplastic compounds
Thermoplastic composites are gaining attention due to their recyclability potential and improved manufacturing processes:
- In-situ polymerization: This technique allows the creation of thermoplastic composites with superior fiber-matrix interfaces. Research at the National Renewable Energy Laboratory (NREL) has shown that this method can reduce manufacturing time by up to 40% compared to traditional thermoset composites.
- Self-repairing thermoplastics: An innovative development in thermoplastic composites is the integration of self-repairing properties. A 2022 article in Advanced Materials reported on a thermoplastic composite that can autonomously repair micro-cracks, which could extend the service life of wind turbine blades by up to 25%.
Biomimetic models in wind turbines
Biomimetics is a science applicable to the design of renewable energy systems2. Currently, the search for greater aerodynamic efficiency in wind turbines has led to innovative developments that are transforming the industry. One of the most promising areas of research is the design of biologically inspired blades, which takes its ideas from nature’s most efficient flyers.
Biomimetics, also known as biomimicry, bioinspiration, or biological inspiration, is a growing field of research that draws inspiration from natural models, systems, and elements to provide innovative design solutions to a variety of problems.
Biomimetic blade technology
Recent studies in biomimetics have focused on replicating the aerodynamic flight properties of birds. Researchers have found that incorporating structures similar to wind turbine blades can significantly improve their performance. The albatross, one of the birds in the study, inspired the researchers because of its unique flight characteristics, especially its dynamics.
Unlike other birds and man-made flying vehicles, albatrosses gain speed and altitude by gliding upwind, reaching speeds in excess of 30 km/h in winds not exceeding 13 km/h3. This ability to generate high aerodynamic lift made the albatross an attractive model for increasing the lift potential of small wind turbines (SWT). The wings of the albatross, characterized by a prominent mid-span flexure and edge, inspired the turbine blade design shown in figure 2.
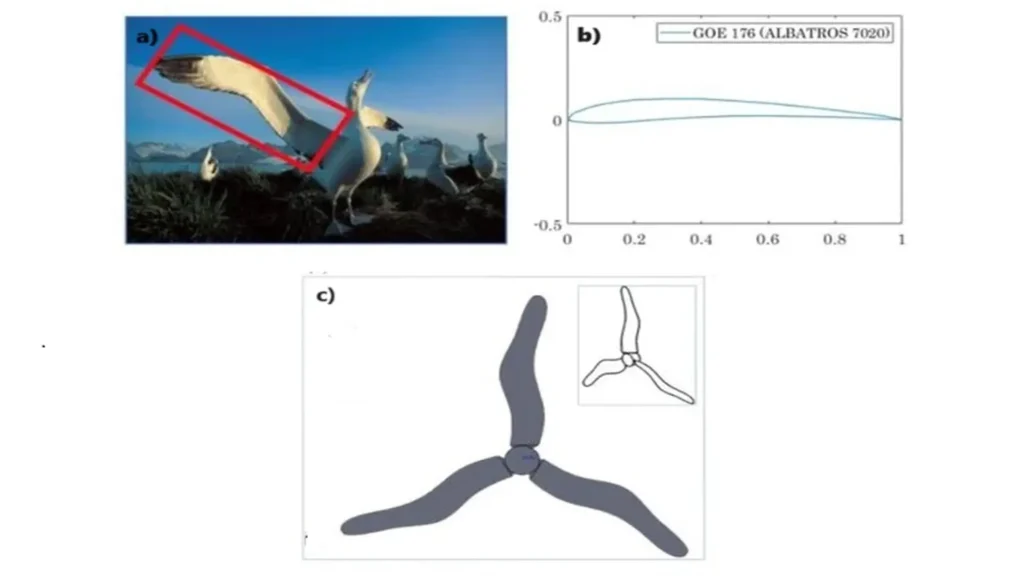
This study demonstrated the potential of bio-inspired SWT designs, particularly those inspired by the golden eagle and albatross, for efficient operation at low wind speeds.
Another 2019 study published in the Journal of Renewable and Sustainable Energy showed that protrusion-inspired blade designs could increase the lift-to-strength ratio by up to 20% compared to conventional smooth-edged blades. This improvement translates into higher energy production and better performance in low wind conditions.
In addition, these biomimetic blades exhibit delayed lift loss characteristics, allowing them to operate effectively over a wider range of wind speeds. The implications of this technology are far-reaching. Not only does it promise increased energy production, but it also opens up possibilities for wind farm development in areas previously considered unsuitable due to inconsistent wind patterns.
The adaptability of these bio-inspired blades could potentially revolutionize the geographical distribution of wind energy production, making it a more universally applicable renewable energy source.
Machine learning in wind energy forecasting
One of the most exciting developments in this field is the application of machine learning algorithms to wind energy forecasting. Traditional weather models, while useful, often fail to predict short-term wind patterns crucial for optimal turbine operation. However, machine learning models can analyze large amounts of historical data along with real-time inputs to provide more accurate and timely predictions.
The implications of these advanced forecasting capabilities are profound. With more accurate short-term predictions, wind farm operators can optimize turbine yaw angles and blade pitch in real-time, maximizing energy capture and minimizing mechanical stress.
In addition, this level of predictability allows for better integration with other energy sources on the grid, reducing the need for backup power and ultimately reducing the overall cost of wind power.
Adaptive control and network stability systems
Another important aspect of smart grid integration is the development of adaptive control systems that can respond to real-time grid conditions. These systems use advanced algorithms to balance power output across multiple turbines, considering factors such as wake effects, grid demand, and potential equipment fatigue.
In addition, these adaptive systems play a crucial role in maintaining grid stability. As the proportion of wind power in the energy mix increases, the inherent variability of wind resources poses challenges for grid operators.
Ongoing research in these areas promises to further improve the reliability and efficiency of wind power, cementing its position as a cornerstone of the global transition to renewable energy sources. As these technologies consolidate, we can anticipate a future in which wind farms are not simply power generators, but intelligent and responsive components of a highly integrated and efficient energy ecosystem.
Environmental benefits of high-performance wind turbines
High-performance wind turbines represent a significant advance in clean energy capture, not only because of their ability to generate electricity more efficiently, but also because of the profound environmental benefits they offer.
Equipped with larger rotor diameters and located at higher altitudes, these turbines are designed to maximize wind utilization while reducing the impact on the land and the environment.
Increasing the size of a turbine from 5 MW to 15 MW, for example, may seem like a purely technical difference, but the environmental implications are considerable. By operating at higher altitudes, these turbines access stronger and more constant winds.
Since the power output of a turbine is proportional to the cube of the wind speed, even a small increase in speed can translate into an exponential increase in energy production. Added to this is the increased swept area of the rotor: a change from 126 meters to 220 meters in blade diameter means that more than three times the energy is captured.
This leap in efficiency translates into a lower number of turbines needed to achieve a given installed capacity. Thus, a 1 GW wind farm, which would require about 200 5 MW turbines, could achieve the same production with only 67 15 MW turbines.
This optimization leads to less land occupation and, therefore, a significant reduction in the alteration of natural habitats.
New technologies in high-performance wind turbines
Another area of focus for improving efficiency is the drive system, the components that convert mechanical energy from the rotor into electrical energy. In some designs, traditional geared drive systems are being replaced by direct drive systems, which eliminate the gearbox altogether. This reduces mechanical losses and maintenance requirements, which could increase overall system efficiency.
Power electronics and intelligent control systems are also key to maximizing turbine efficiency. Modern wind turbines use sophisticated algorithms to continuously adjust their operation based on wind conditions, grid demands, and component conditions. These systems can optimize energy production, reduce wear and tear, and even predict and prevent potential failures.
A particularly exciting development in the search for high-efficiency wind turbines is the emergence of floating offshore wind technology. These turbines can be installed in deep water, giving them access to some of the strongest and most consistent wind resources on the planet. While still in the early stages of commercial deployment, floating wind turbines have the potential to significantly increase global wind power generation capacity.
It is good to note that the efficiency of a wind turbine is not only about its maximum output but also about its ability to generate power in a wide range of wind conditions. High-efficiency turbines are designed to have a low start-up speed (the minimum wind speed at which they start generating power) and a high capacity factor (the ratio of actual power output to the maximum possible output over time).
Conclusions
The advancement of technologies such as multi-rotor turbines and airborne systems demonstrates the potential of wind energy to access more powerful energy resources, marking a path towards greater efficiency in renewable energy generation.
The integration of advanced aerodynamics, materials science, and control systems in high-efficiency wind turbines underscores the human capacity to innovate in the quest for a more sustainable energy future.
The combination of technological advances in wind energy and global political commitment is key to positioning wind energy as a central pillar in the fight against climate change, contributing significantly to a more sustainable and balanced global energy system.
References
- https://www.enelgreenpower.com/es/learning-hub/energias-renovables/energia-eolica/aerogenerador
- The first electric superconducting generator for medium power wind turbines
- Yossri, W.; Ben Ayed, S.; Abdelkefi, A. Evaluation of the Efficiency of Bioinspired Blade Designs for Low-Speed Small-Scale Wind Turbines with the Presence of Inflow Turbulence Effects. Energy 2023, 273, 127210. [Google Scholar] [CrossRef]