Introduction
The science of advanced energetic materials touches every aspect of human life, from renewable energy, to energy efficiency, nanotechnology, health, construction, transportation, manufacturing processes, recycling and much, much more. With their help, we can protect the environment and ensure a brighter future for generations to come.
Advanced energy materials are designed to efficiently convert, store and manage energy in a variety of applications. These materials include metals, polymers, ceramics and composites designed for superior performance in energy generation, storage and conservation.
That is why the aim of this article is to disseminate information on the use of advanced energy materials; and on innovative and improved devices to drive the transition to greener technologies and contribute to a sustainable future.
What are advanced energy materials?
Advanced energy materials are fundamental to the development of sustainable energy technologies, such as solar panels and high-efficiency batteries. These materials, which include perovskites, graphene and nanomaterials, offer improvements in the efficiency, durability, and cost of energy devices. Ongoing research in this field seeks to innovate solutions to mitigate climate change and increase accessibility to renewable energy sources.
This is also defined as materials designed to optimize energy generation, storage, and use in the global energy transition, including renewable energy, storage systems and energy conversion technologies.
Main categories of advanced energetic materials
- Energy storage materials:
- Solid-state batteries: Improve safety and energy density.
- Super-capacitors: Advanced materials such as graphene for high charging and fast discharge capacity.
- Metal hydrides: Hydrogen storage solutions.
- Photovoltaic materials:
- Perovskites: High efficiency and potential for low-cost manufacturing.
- Multi-junction cells: Increase efficiency by taking advantage of different wavelengths.
- Thermoelectric materials:
- Tellurium-based alloys: Efficient conversion of waste heat into electricity.
- Zintls composites: Promising materials due to their low thermal conductivity.
- Materials for green hydrogen:
- Advanced electrocatalysts: Materials such as platinum or metal oxides for efficient water electrolysis.
- Proton conducting membranes: Improve fuel cell efficiency.
- Carbon capture and storage materials:
- MOFs (metal-organic frameworks): High capacity to trap CO2 at molecular level.
- Regenerative sorbents: Adapted for industrial applications.
- Superconducting materials:
- Applications in lossless power transmission and fusion reactors.
Propiedades de los materiales avanzados
Advanced materials are fundamental in the technological revolution, offering improvements in efficiency and sustainability. In the energy sector, they play a crucial role not only for their generation capacity but also for their storage. They enable a wide variety of applications that benefit from their characteristics.
These materials exhibit outstanding properties that make them ideal for energy applications. Among the most important are:
- Electrical conductivity: The ability to conduct electricity efficiently, crucial for batteries and supercapacitors.
- Thermal conductivity: Properties that allow heat to be handled efficiently, essential in solar panels.
- Mechanical strength: Materials such as graphene offer high strength with low weight, useful in load-bearing structures and energy leagues.
- High energy density: Required for portable applications and mass storage.
- Chemical stability: Crucial for extreme conditions.
- Sustainability: Use of abundant materials and low environmental impact manufacturing processes.
These properties are investigated to optimize energy transfer, which is of vital importance in the development of more efficient technologies.
What are the advances in advanced materials for solar energy?
Advances in materials include three key areas: nanomaterials, such as graphene, carbon nanotubes and quantum dots, which stand out for their unique properties at the nanometer scale; emulating natural structures; and hybrid technologies, which integrate combinations of materials to maximize efficiency in various applications.
Nanomaterials, Bioinspired materials and hybrid technologies
Nanomaterials: Advances and applications
Nanomaterials have transformed the field of advanced energetic materials due to their unique properties derived from their nanometer scale. These properties include high area-to-volume ratio, quantum behavior and molecular customization capabilities. Some key developments include:
1- Graphene and its derivatives: graphene, a two-dimensional material composed of a single layer of carbon atoms, has shown enormous potential in energy applications due to its exceptional electrical and thermal conductivity.
- Super-capacitors: Graphene’s structure allows it to store large amounts of charge, making it ideal for devices that require fast charge and discharge cycles.
- Battery electrodes: Its integration in lithium-ion batteries significantly improves energy density and lifetime.
- Energy conversion: In solar cells, it is used as a transparent conductive layer, improving conversion efficiency.
2. Nanotubos de carbono (CNTs): Estos materiales 1D presentan una resistencia mecánica y conductividad eléctrica extraordinarias.
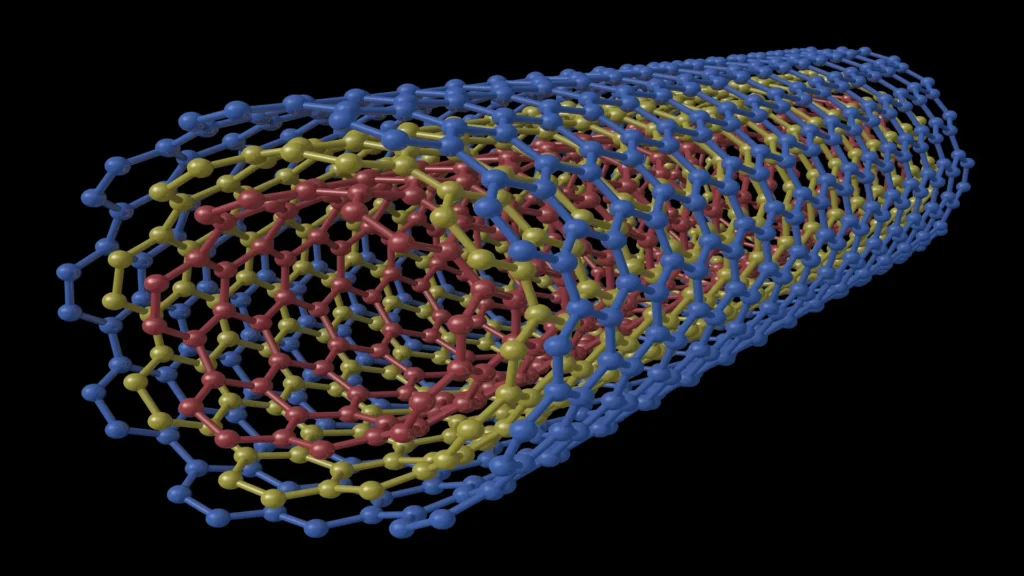
- Fuel cells: CNTs act as supports for catalysts, increasing the active surface area and durability.
- Hydrogen storage: Thanks to their high adsorption capacity, they are being investigated for hydrogen-based energy systems.
3. Quantum Dots: These semiconductor nanocrystals allow highly efficient and tunable light absorption and emission by design.
Perovskite solar cells: Incorporating quantum dots improves light capture and reduces energy losses.
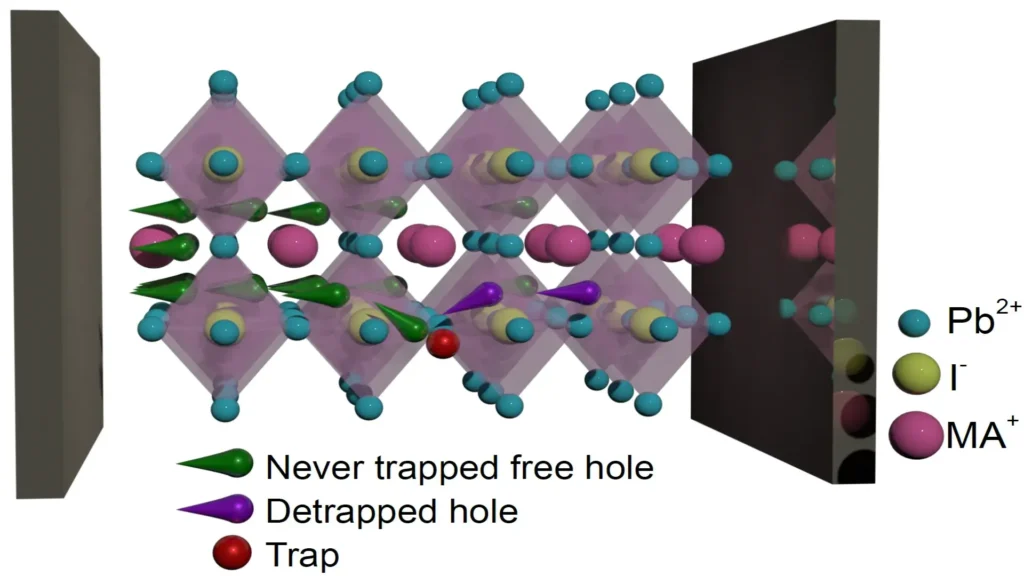
Recent advances include the development of hybrid perovskites, which promise higher efficiency and lower cost; flexible organic materials for curved surface applications; nanomaterials such as quantum dots to enhance light absorption; and thin films that offer lightness and versatility in their integration into different surfaces.
4. MOFs (Metal-Organic Frameworks): These porous structures with extremely high surface area are redefining energy capture and storage.
- CO2 capture: Chemically functionalized MOFs can selectively trap CO2 molecules, improving efficiency in industrial processes.
- Electrolytes in batteries: Used as matrices to stabilize ion transport.
Technologically bio-inspired materials
Bio-inspired materials represent a revolutionary approach to the design of technological solutions by emulating properties and mechanisms present in nature. Inspired by the characteristics of living organisms, such as strength, flexibility and self-repair capacity, these materials combine natural functionality with scientific innovations to improve applications in key sectors. The ability to replicate biological structures enables the development of lighter, more durable and sustainable materials, responding to the needs of a wide variety of industries.
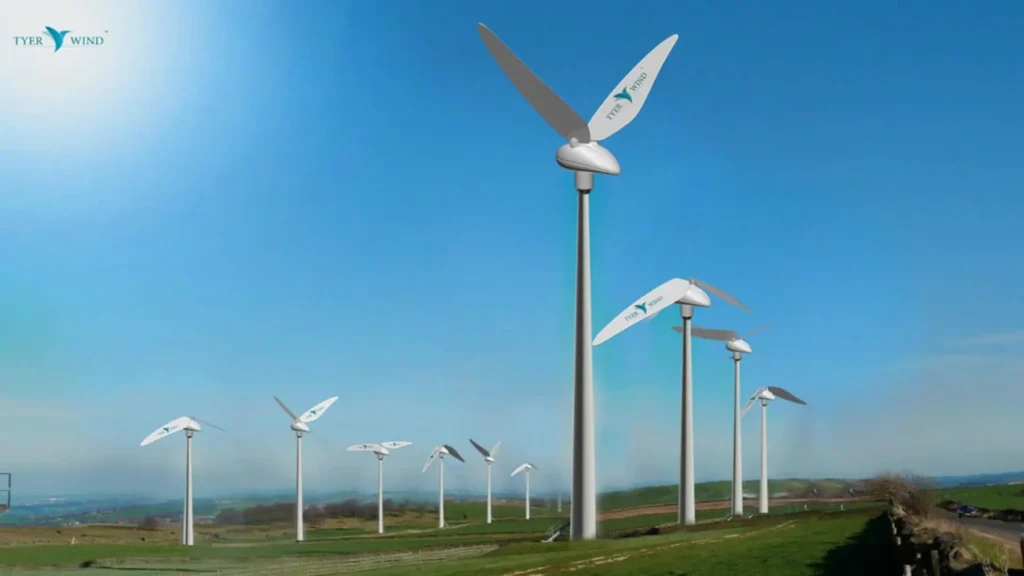
Bioinspiration research is not only focused on copying natural structures, but also on understanding the biological processes that give rise to them. This in-depth knowledge facilitates the creation of advanced materials, with unique properties that can transform fields such as medicine, through more effective devices; energy, with more efficient storage and conversion materials; or construction, through more resistant and environmentally friendly structures. Thus, bioinspired materials are positioned as an essential tool in the development of future technologies.
Hybrid technologies: Synergies between materials
Combining materials with complementary properties has led to hybrid technologies that maximize efficiency and functionality in energy applications.
1. Hybrid battery systems: Integrating advanced materials into traditional batteries is expanding energy storage capabilities:
- Baterías litio-azufre: Al combinar ánodos de silicio con recubrimientos de grafeno, se logra una alta capacidad energética y menor degradación.
- Baterías de estado sólido híbridas: Combinan electrolitos de cerámica y polímeros para mejorar la seguridad y la densidad energética.
2. Hybrid photovoltaic conversion technologies: These technologies take advantage of multiple mechanisms to capture and convert solar energy:
- Thermal-photovoltaic solar cells: Integrate thermoelectric materials with perovskites to capture both light and solar heat, improving overall efficiency.
- Multifunctional coatings: Nanocomposite polymers that act simultaneously as anti-reflective and conductive filters.
3. Biohybrid materials: Inspired by biological systems, they combine synthetic materials with biomaterials for storage and conversion applications:
- Artificial photosynthesis: Semiconductor materials coupled with natural enzymes to generate solar fuels.
- Advanced membranes: Mixtures of biopolymers and MOFs to improve gas separation in industrial processes.
4. Hybrid energy devices:
- Super-capacitor-batteries: Integrate asymmetric electrodes to combine high energy density and high power output.
- Hybrid fuel cells: Incorporate bifunctional catalysts for oxidation and reduction in a single system.
Impact of these innovations
The adoption of nanomaterials, bio-inspired materials and hybrid technologies is redefining the energy materials landscape, enabling more efficient, sustainable and adaptable solutions for future applications. These developments are also accelerating the path to cleaner economies and resilient energy systems.
Advantages of advanced energy materials
- Energy efficiency: Improve the performance of energy storage and conversion systems, leading to lower energy consumption and greater sustainability.
- Renewable energy: These materials improve the efficiency and reduce the cost of renewable energy technologies such as solar panels and wind turbines, promoting wider adoption of clean energy sources.
- Innovation: The development of advanced energy materials drives technological advances in several sectors, including batteries, fuel cells and super-capacitors.
- Economic growth: Research, development and manufacturing of these materials stimulate job creation and economic activity in the renewable energy sector.
- Environmental impact: By enabling more efficient and cleaner energy technologies, advanced energy materials contribute to reducing dependence on fossil fuels and mitigating climate change.
Advanced energy materials have a crucial role to play in the development of emerging technologies for the decarbonization of the power grid, especially in the challenging “last 10%” of emissions. These materials can significantly support clean hydrogen production, advanced nuclear power and carbon capture and storage.
Advanced ceramic materials
In recent years, the materials science community has shown increasing interest in nanostructured materials due to their unique physical and chemical properties determined by quantum size effects. Within this field, advanced ceramic materials, such as perovskites, stand out for a wide range of properties, including ionic conductivity, superconductivity, magnetoresistance and ferroelectricity.
These properties perovskites, especially alkaline earth perovskites, key materials in applications such as electrical ceramics, solid oxide fuel cells, refractory materials and hydrogen sensors.
- Crystalline structure of perovskites: Detailed description of their fundamental characteristics and structural flexibility.
- Advanced synthesis methods: Comparison of conventional and emerging techniques to optimize their properties.
Perovskite-type oxides, thanks to their flexible crystal structure, allow the incorporation of ions that optimize their photocatalytic properties. This positions them as potential candidates to replace TiO₂, the most widely used commercial photocatalyst today. Traditionally, perovskites are synthesized by solid-state reactions, which can compromise their structural properties and, consequently, the efficiency in photocatalytic reactions.
To overcome these limitations, alternative synthesis methods have been developed, such as sol-gel, microwave, electrospinning, hydrothermal and solvothermal methods, and complex polymerization. These approaches have led to significant improvements in the structural and functional quality of these materials. In the global context, the search for sustainable alternatives for renewable energy generation, more efficient devices and advanced technologies for soil, water and air remediation has consolidated perovskites as a promising option.
Conclusions
Advanced energy materials are critical to addressing the ultimate challenge of complete power grid decarbonization. Their development and application in emerging technologies such as clean hydrogen production, advanced nuclear power and carbon capture can provide the solutions needed to achieve net-zero emissions targets.
Advanced energy materials play a key role in addressing global energy challenges and paving the way to a more sustainable future by improving energy production, storage and conservation in a variety of applications.
The production of certain renewable energy technologies requires a significant increase in critical materials. For example, demand for battery materials such as graphite, lithium and cobalt is expected to increase by more than 450 % over 2022 levels by 2050.
References
- https://college.unc.edu/2024/11/traps-perovskite/
- https://www.energy.gov/eere/solar/perovskite-solar-cells
- https://www.powerelectronicsnews.com/overcoming-the-materials-challenge-for-renewable-energy/
- https://www.tms.org/portal/downloads/publications/studies/MaterialsandEnergyEfficiency/1005AdvMatlsforEnergy.pdf
- https://theconversation.com/nuevos-materiales-para-convertir-las-depuradoras-de-aguas-residuales-en-fabricas-de-hidrogeno-verde-231649