Introduction
For structural engineering in seismically active areas, the protection of buildings and infrastructure against the devastating forces of earthquakes represents a constant challenge. While traditional methodologies focused on strengthening structures to resist these loads, the concept of seismic isolation has revolutionized the approach. This not-so-innovative technique seeks to decouple the superstructure from ground motion by interposing flexible elements that filter and significantly reduce the transmission of seismic energy.
And it was called not so innovative because in ancient constructions, especially in ancient Mesopotamia, it is known that temples were built with a dome at the top and another of the same characteristic at the base, which assimilated all the horizontal oscillatory seismic movement of an eventual catastrophe. However, man in his determination to reach the sky, every day builds buildings that are a risk if the proper precautions are not taken to avoid their collapse caused by large-scale earthquakes.
Within this scenario, natural rubber dampers are now emerging as key components, offering versatile solutions to mitigate seismic risks. Among them, the Linear Natural Rubber (LNR) damper and the Lead Core Rubber (LRB) damper represent two distinct but complementary strategies to achieve structural resilience.
Linear Natural Rubber (LNR): Insulation with flexibility
The Linear Natural Rubber (LNR) damper, exemplified by models such as the LNR 700-II, is one of the most frequently used devices in building construction, based on the remarkable ability of laminated natural rubber with steel plate inserts to provide horizontal flexibility and vertical stiffness simultaneously (Skinner et al., 1993).
Due to its multilayer construction, the device can support the considerable weight of the superstructure thanks to the vertical stiffness of the steel plates, while the low horizontal stiffness of the rubber facilitates large lateral deformations during a seismic event.
That is, while the weight of the building by gravity tends to press downward, the device assimilates the pressure, while the lateral oscillatory or side-to-side or horizontal movement, as you want to call it, dampens and balances the stresses. Therefore, this flexibility is fundamental to extend the natural vibration period of the structure, moving it away from the range of frequencies that predominate in earthquakes and thus reducing the accelerations transmitted to the building.
The natural rubber used is exclusively selected for its elasticity, durability and the stability of its mechanical properties. The steel plates, on the other hand, ensure that this device can have an even distribution of loads to prevent bulging of the rubber. It is also important here to talk about the vulcanization process that creates a strong bond between these materials.
While the maximum load capacity of the specific LNR 700-II requires manufacturer’s data, LNR bearings in general can support loads ranging from a few tons to several thousand, depending on their design.
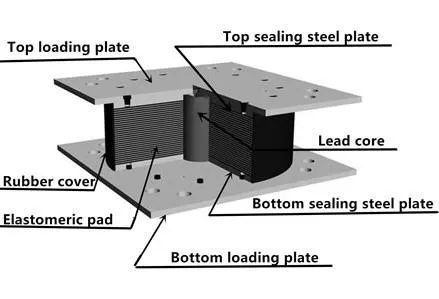
A noteworthy aspect of LNR is its inherent low damping, typically between 2% and 5% of the critical damping. This means that, while effective for isolation, it requires the incorporation of additional damping devices (such as viscous or hysteretic dampers) to control excessive lateral displacements that could occur during severe earthquakes.
The relatively linear behavior of natural rubber within the seismic deformation ranges facilitates the analysis of the system, and its re-centering capability helps the structure to return to its original position after the event.
Lead Rubber Bearing (LRB): Integrating insulation and cushioning
In contrast, the Lead Rubber Bearing (LRB) introduces a different strategy by integrating the damping function directly into the bearing. Its design is also based on layers of natural rubber vulcanized to steel plates, but is distinguished by the presence of a cylindrical lead core inserted vertically through the center of the bearing.
During an earthquake, lateral deformation of the LRB support causes plastic deformation of the lead core. This plastic deformation process dissipates a significant amount of seismic energy through the hysteretic damping, resulting in a much higher effective damping compared to the LNR (typically between 10% and 30% of the critical damping or even higher).
This integrated damping capability often allows the structure’s displacements to be effectively controlled without the need for additional external dampers, simplifying design and potentially reducing costs.
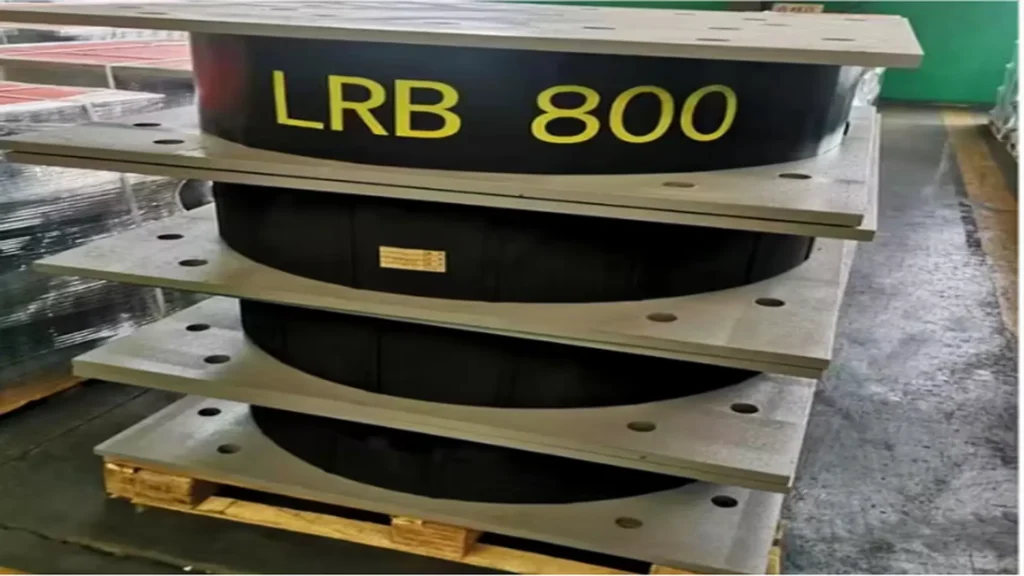
However, the inclusion of the lead core introduces nonlinear behavior in the LRB due to the plasticity of the lead. The core has a relatively low creep strength, which means that it begins to dissipate energy even with moderate seismic motions. Like the LNR, the LRB also provides isolation by extending the natural period of the structure and offers significant vertical load capacity, determined by its dimensions and construction.
Implementation and economic benefits: A global perspective
The implementation of seismic isolation systems with natural rubber binders, both LNR and LRB, has grown significantly in seismically active regions around the world. Countries such as Japan, the United States, New Zealand, Italy and Chile have adopted these technologies in a wide range of structures, from residential buildings to hospitals, bridges and critical data centers (Buckle et al., 2002).
While exact implementation figures for the specific LNR 700-II model are difficult to obtain without proprietary data, LNR technology in general has been widely used, often in combination with external dampers. On the other hand, the LRB has gained popularity for its ability to integrate isolation and damping in a single device, which can be advantageous in terms of design and space.
The following video shows an animation of the operation of a seismic protection system. Source: Mageba Group.
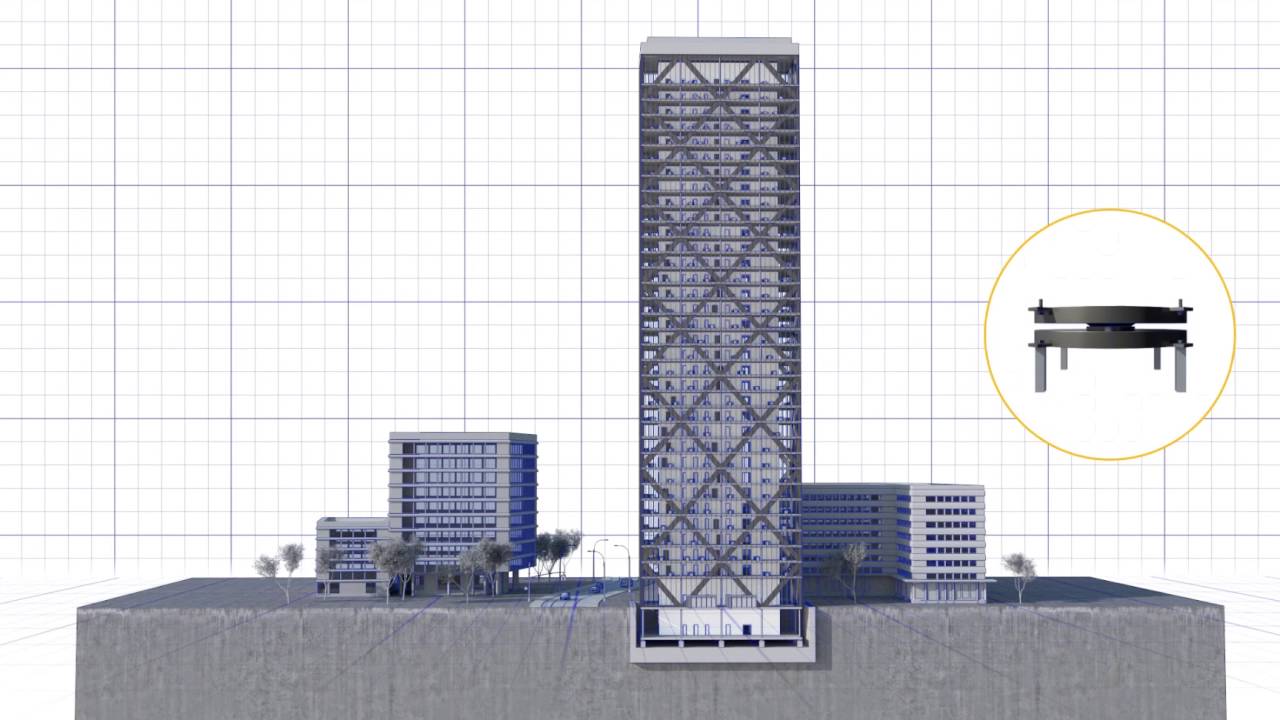
Seismic protection systems.
From the point of view of the project formulator and designer, the investment in this type of supplements has a favorable impact on the economics of the project in the prognosis of long-term scenarios. The benefits of seismic isolation are increasingly evident and tangible. These devices integrated in their construction suffer significantly less structural damage during earthquakes, which translates into lower repair and reconstruction costs (EERI, 1995), and studies carried out in different tests have confirmed this.
It is also important to note that the protection of valuable contents in buildings such as hospitals and museums also represents a substantial economic saving. On the other hand, the ability to maintain operational safety in affected buildings after an earthquake is invaluable. The builder will probably think that the initial investment in seismic isolation may be more than the budgeted cost of the project, but the long-term savings in terms of damage reduction, content protection and operational continuity far outweigh these costs.
The strategic choice: LNR versus LRB
The decision to use LNR or LRB dampers in a seismic isolation project depends on a number of specific factors:
- Damping requirements: If a high level of damping is required to control displacements at a site with high seismicity, the LRB may be a more compact and efficient solution by integrating this function. If the required damping is lower or can be easily provided by external devices, the LNR may be a viable option.
- Space constraints: In projects where space for the installation of additional dampers is limited, the LRB offers an advantage by consolidating the isolation and damping functions.
- Cost considerations: The initial cost of LNR supports may be lower, but the total system cost may increase with the addition of dampers. LRBs may be more expensive individually, but could be more economical by eliminating the need for separate damping devices.
- Desired structural behavior: LNR linearity may be preferable in certain structural analyses, while LRB nonlinearity should be modeled appropriately.
Feature | LNR (Linear Natural Rubber Bearing) | LRB (Lead Rubber Bearing) |
Damping | Low inherent damping (2-5%). Generally requires additional dampers. | High effective damping (10-30% or more) due to lead core. May not require additional dampers. |
Damping mechanism | Mainly through external devices. | Plastic deformation of the lead core (hysteretic damping). |
Performance | More linear. | Nonlinear due to lead plasticity. |
System complexity | It can be more complex due to the need for separate dampers. | It can be simplified by integrating isolation and damping in a single device. |
Initial cost | LNR supports may be less expensive, but the total cost of the system may increase with additional shock absorbers. | LRB supports can be more expensive individually. |
Typical applications | Structures where significant isolation is required, and additional dampers can be accommodated. | Structures where a compact solution with integrated insulation and damping is sought, or where space for dampers is limited. |
Conclusions
Both Linear Natural Rubber (LNR) dampers, exemplified by the LNR 700-II, and Lead Core Rubber (LRB) dampers represent significant advances in seismic isolation engineering. The LNR offers a robust solution for isolation, often requiring supplementation with external dampers, while the LRB effectively integrates isolation and damping in a single device.
The choice between the two will depend on the specific needs of each project, considering site seismic characteristics, damping requirements, space constraints and economic considerations. As the understanding of seismic risks evolves, implementation and innovation in isolation technologies such as natural rubber dampers will continue to be critical to building safer, more resilient and economically sustainable structures in an earthquake-prone world.
References
- Buckle, I. G., Mayes, R. L., & Kelly, J. M. (2002). Seismic isolation: History, application, and outlook. Earthquake Spectra, 18(1), 3-26.
- Earthquake Engineering Research Institute (EERI). (1995). Northridge Earthquake of January 17, 1994: Reconnaissance Report. EERI Publication No. 95-03.
- Naeim, F., & Kelly, J. M. (1999). Design of seismic isolated structures. John Wiley & Sons.Skinner, R. I., Robinson, W. H., & McVerry, G. H. (1993). An introduction to seismic isolation. John Wiley & Sons.