Table of Contents
- Introduction
- What are synthetic polymers?
- Types of synthetic polymers
- Functionalities of synthetic polymers
- Environmental impacts of synthetic polymers
- Technological innovations for the sustainability of synthetic polymers
- Balance between functionality and sustainability
- Innovative applications of sustainable polymers
- Conclusions
- References
Introduction
Synthetic polymers, present in everyday life, are macromolecular materials designed to offer specific functionalities in various applications. From food packaging to electronic components, their versatility and adaptability have made them indispensable in industry and consumption. However, their production and final disposal have generated great environmental concerns, driving the search for more sustainable alternatives.
This article discusses the current landscape of these materials, addressing both their functionalities and the environmental issues they raise, and highlighting technological innovations that focus on balancing the performance of these materials with the need for ecological sustainability.
What are synthetic polymers?
Synthetic polymers are macromolecules formed by the polymerization of monomers, i.e. the repeated joining of smaller structural monomer units by means of covalent bonds; they are divided into two categories according to their behavior when heated: thermoplastics and thermosets. These are synthesized through controlled polymerization processes, such as addition or condensation polymerization, under specific conditions in laboratories or industrial plants.
Unlike natural polymers, such as starch or proteins, synthetic polymers are synthesized with specific properties. The monomers used for their synthesis come mainly from non-renewable sources, such as petroleum, and their versatility allows the manufacture of materials with different applications, ranging from plastics to synthetic fibers, adaptable to various shapes through molding or extrusion processes.
Main characteristics
- Composition: Chains of monomers linked by covalent bonds.
- Versatility: Their properties can be modified during synthesis to adapt them to various needs.
- Functionalities: Mechanical strength, flexibility, elasticity, electrical and thermal insulation, chemical resistance, transparency, gloss and biodegradability.
Types of synthetic polymers
- Polyvinyl chloride (PVC): Its components are derived from sodium chloride, natural gas or petroleum, and include chlorine, hydrogen and carbon; they are used in pipes, coatings and windows.
- Polyethylene (PE): Also known as polymethylene, it is the simplest of the polymers from the chemical point of view, composed of a linear and repetitive hydrogen unit. It is one of the most widely used plastic materials in the world and is used in packaging, bags and films.
- Polypropylene (PP): The lightest and most crystalline, it is also the newest of the plastics being manufactured on a large scale. It is susceptible to degradation by light, heat and oxygen. It is present in food packaging, textiles and auto parts.
- Polystyrene (PS): A thermoplastic, linear, amorphous, transparent polymer used in food packaging, toys and insulation.
- Polyethylene terephthalate (PET): A polymer obtained chemically by the polycondensation reaction between terephthalic acid and ethylene glycol, it belongs to the group of synthetic materials called polyester. It is used in beverage bottles, textile fibers and films.
- Nylon: Used in textiles, ropes and industrial components.
The following image shows the molecular structure of polystyrene (PS): Thermoplastic polymer obtained from the polymerization of the monomer styrene.
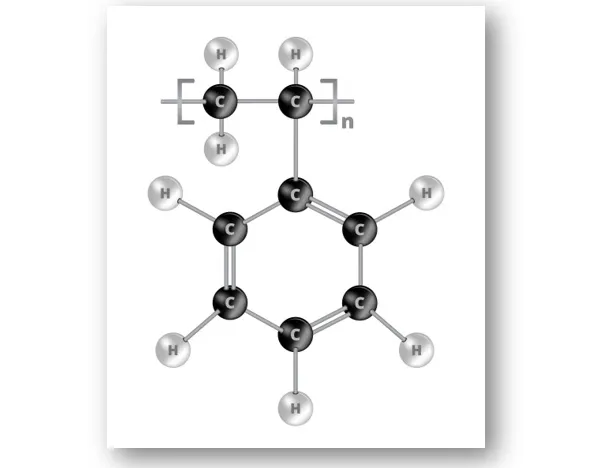
Functionalities of synthetic polymers
Synthetic polymers are characterized by their molecular structure composed of repeating chains of monomers. This structure gives them properties that can be modulated during their synthesis to adapt them to various applications. Some of the most outstanding functionalities are:
- Mechanical resistance: polymers can be designed to resist tensile, compressive or impact stresses, thanks to their covalent bonds and crystalline or amorphous structures. Examples such as high-density polyethylene (HDPE) or aramid polymer (Kevlar) are used in structural applications such as automotive components, helmets or in the aerospace industry due to their high mechanical strength.
- Flexibility and elasticity: Some polymers exhibit elastic behavior, deforming under stress and recovering their original shape after the release of force, due to the nature of their molecular chains. Elastomers such as polybutadiene (BR) or ethylene-propylene-diene polymer (EPDM) are commonly used in the manufacture of tires, sealants and adhesives.
- Electrical and thermal insulation: Polymers such as polyethylene (PE) or polypropylene (PP) have low thermal and electrical conductivity due to their non-polar molecular structure, making them ideal materials for cables, electrical insulation and coatings in the electronics and construction industries.
- Chemical resistance: Some polymers show high chemical inertness to aggressive substances due to their stable chains and strong bonds. Polytetrafluoroethylene (PTFE), known as Teflon, and polypropylene (PP) are examples that are used in the manufacture of chemical containers, pipes and protective coatings in the chemical industry.
- Transparency and gloss: Polymers, such as polymethylmethacrylate (PMMA) and polycarbonate (PC), have structures that allow light transmission, providing high transparency and gloss. These materials are commonly used in the manufacture of lenses, windows or food and beverage containers.
- Biodegradability: Some synthetic polymers, such as polyhydroxyalkanoate (PHA) or polylactic acid (PLA), are designed to biodegrade under specific environmental conditions, which reduces their persistence in the environment and facilitates their management as waste compared to conventional polymers.
Environmental impacts of synthetic polymers
Despite their numerous functionalities, their production and uses have generated a series of environmental problems that require urgent attention:
- Dependence on fossil resources: most of these polymers are derived from fossil hydrocarbons, such as petroleum and natural gas. The extraction and refining processes of these resources contribute to the release of greenhouse gases (GHG) such as carbon dioxide (CO₂), and also drive climate change due to emissions generated in the life cycle of petrochemicals.
- Persistence in the environment: Synthetic polymers, such as polyethylene or polypropylene, are highly resistant to biodegradation due to their stable molecular structures. This resistance causes them to persist for centuries in ecosystems, accumulating in soils, water and oceans, contributing to environmental pollution and generating risks for biodiversity.
- Microplastics: The mechanical decomposition and photodegradation of these materials in the environment produces microplastics, microscopic polymer particles that can be ingested by marine and terrestrial organisms. These microplastics accumulate in the food chain, affecting the health of living beings and potentially impacting human health through indirect ingestion of contaminants adsorbed on their surface.
- Difficulty of recycling: Structural heterogeneity, combined with the incorporation of additives and plasticizers, makes recycling difficult. This limits the regeneration of polymers through mechanical or chemical recycling processes, increasing the production of new polymers from monomers derived from fossil resources. This linear production and disposal cycle contrasts with the principles of the circular economy, which seek to maximize the reuse of materials and reduce dependence on non-renewable resources.
Technological innovations for the sustainability of synthetic polymers
Faced with the environmental challenges associated with these materials, the scientific community and industry have intensified research and development of more sustainable technologies. Some of the most promising innovations include:
- Bio-based polymers: Polymers are being developed from renewable sources, such as starch, cellulose, vegetable oils or even agricultural residues. One example is polylactic acid (PLA), which is produced from the fermentation of corn starch. These polymers are biodegradable under industrial composting conditions, and also have a more sustainable life cycle.
- Biodegradable polymers: Synthetic polymers are being synthesized that break down under specific environmental conditions, such as industrial composting or anaerobic digestion. Polymers such as polyhydroxyalkanoate (PHA), produced by microorganisms from organic sources, and polycaprolactone (PCL), which biodegrades rapidly, can reduce plastic waste accumulation and microplastic pollution by being biodegradable without generating toxic products.
- Chemical recycling: This is an area of great interest, as it allows polymers to be broken down into their original monomers, which are then used to synthesize new polymers. One example is the recycling of polyethylene terephthalate (PET) through a hydrolysis process, which breaks down the polymer into its monomers (terephthalic acid and ethylene glycol), which can be reused. This technology could increase the recycling rate and reduce the need for new fossil resources.
- Circular polymer design: The development of polymers that are easily recyclable, with structures that allow continuous reuse in multiple life cycles, is being promoted. An example of this is the development of thermoplastic polymers, such as polyethylene (PE) and polypropylene (PP), which can be melted and reshaped without losing their properties.
- Use of sustainable additives and fillers: Researchers are studying sustainable alternatives to conventional additives and fillers used in polymer manufacturing. Focusing on the use of silica nanoparticles or recycled carbon fillers to improve mechanical and thermal properties without relying on petroleum-based synthetic additives. In addition, the use of biomaterials such as cellulose or chitin-based fillers, which could replace conventional plastics without compromising material performance, is being investigated.
Balance between functionality and sustainability
Achieving a balance between the functional properties and sustainability of synthetic polymers represents a challenge in the production of these materials. While traditional polymers offer characteristics such as durability, versatility and efficiency in various applications, these properties are often achieved at the cost of high environmental impact. However, recent advances aim to develop solutions that do not compromise on performance, but do integrate sustainability principles.
This includes the adoption of materials of renewable origin, such as those derived from biomass or organic waste, which reduce dependence on fossil resources. In addition, design for recycling and improved chemical and mechanical recycling processes are enabling these materials to be reused without losing their functional properties. Thus, advances in next-generation synthetic polymers seek to maximize resource efficiency and minimize waste generation, fostering a circular economy that promotes reuse and minimizes ecological impact.
Find out how plastics recycling faces challenges and how waste can be turned into new products. In this video, Professor Kim Ragaert and her team at Maastricht University explain the difficulties of plastic recycling and how they are working to overcome these challenges, contributing to the circular economy.
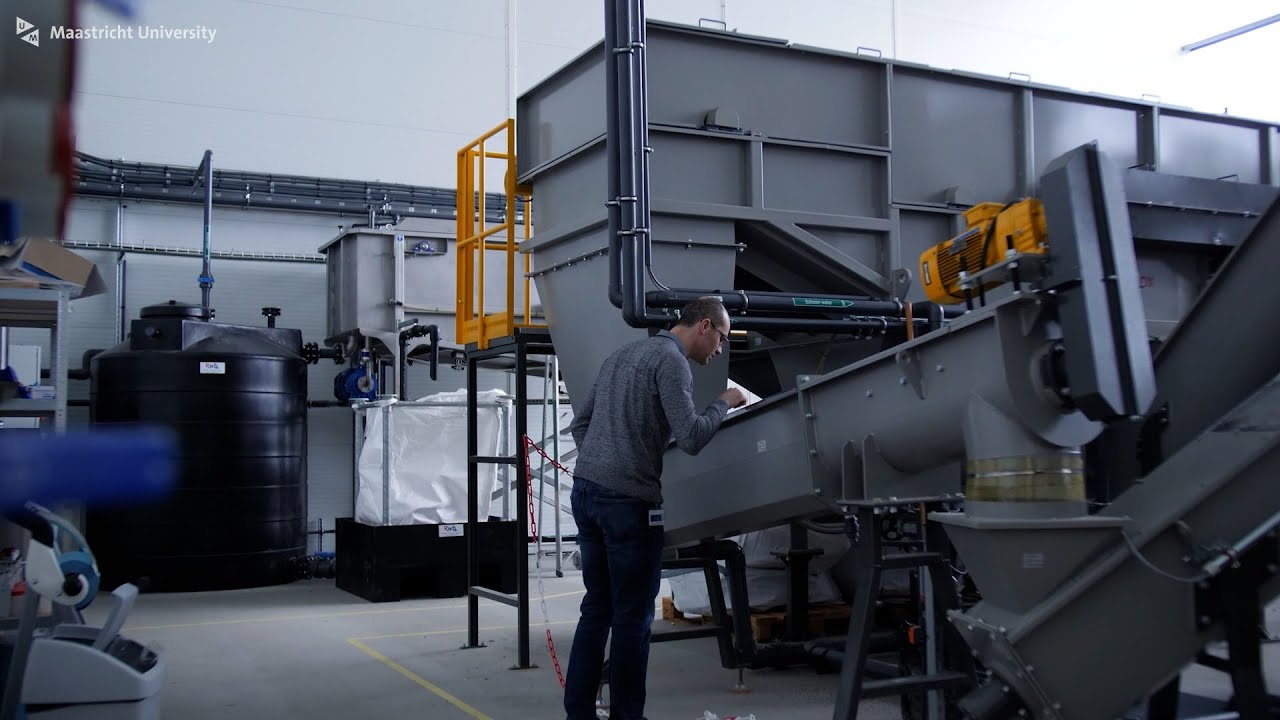
Recycling of synthetic polymers, making circular plastics.
Innovative applications of sustainable polymers
Sustainable polymers are being used in a variety of innovative applications. A prime example is the use of biodegradable polymers in the manufacture of food packaging. Companies such as Danimer Scientific have developed Nodax™ PHA, a biodegradable polymer derived from vegetable oils, which decomposes under natural conditions without generating toxic residues.
This material is used to produce food product packaging that, in addition to being completely biodegradable, reduces the environmental impact of traditional plastics. The technology promotes circularity, since, being biodegradable, the product does not contribute to contamination by microplastics, which helps to close the product’s life cycle.
Conclusions
Synthetic polymers have transformed our daily lives, offering new properties that make them indispensable for various industrial and commercial applications. However, their production and final disposal have generated considerable environmental impacts; therefore, there is an urgent need to search for more sustainable alternatives. Fortunately, scientific and technological advances are opening new perspectives to reconcile the functionality of polymers with the principles of sustainability.
The development of bio-based polymers, improved recycling processes and design for reuse are fundamental steps to reduce dependence on fossil resources and minimize the ecological footprint of these materials. By adopting circular economy strategies and optimizing the life cycle of polymers, it is possible to maintain their versatility and performance without compromising environmental health. In this way, the synthetic polymers of the future will be able to contribute to a more responsible and less impactful development for global ecosystems.
References
- https://prezi.com/p/fgtoxqs930ly/los-polimeros/
- https://www.uniuso.com/blog/glosario/polimero-sintetico/